Wikipedia:Reference desk/Archives/Science/2008 December 22
Science desk | ||
---|---|---|
< December 21 | << Nov | December | Jan >> | December 23 > |
Welcome to the Wikipedia Science Reference Desk Archives |
---|
The page you are currently viewing is an archive page. While you can leave answers for any questions shown below, please ask new questions on one of the current reference desk pages. |
December 22
[edit]Generator
[edit]Why is copper used in generators instead of other metals? —Preceding unsigned comment added by 65.5.174.233 (talk) 01:29, 22 December 2008 (UTC)
- Because copper has a high electrical conductivity. Among (relatviely) common metals, copper is one of the most conductive around. Silver is somewhat more conductive, but also prohibitively more expensive. Copper is also one of the most ductile metals, making it well suited for making wire. --Jayron32.talk.contribs 01:58, 22 December 2008 (UTC)
- During the second world war the Manhatten Project needed to make large electrical machines for extracting uranium isotopes to make the atom bomb. Copper was in extremely short supply because the sources were cut off and the demand for military applications was high. So the US Mint had to turn over all of its silver bullion stocks to make the cabling the project needed. So I guess the second most suitable "commonly found" metal is indeed silver. SteveBaker (talk) 14:09, 22 December 2008 (UTC)
- Alumin(i)um is a third choice, and it probably has a larger installed base than pure silver wiring. While not used extensively in generator windings (as far as I know) aluminum wire is widely employed in utility transmission lines; it was also popular in residential construction during a spike in copper prices in the 1960s and 70s. TenOfAllTrades(talk) 14:41, 22 December 2008 (UTC)
- I expect that a generator could be made with the stator and rotor conductors made of various metals other than copper and it could work ok. Probably even mercury could be used, if confined in strong enough tubing. Cost/weight/size/strength are the design factors which suggest copper. Consider superconductive windings, which would not be copper. Edison (talk) 20:18, 22 December 2008 (UTC)
- I seem to remember hearing that silver is the best conductor among wires of the same thickness, copper is best with respect to weight, and aluminum is next best either way. —Tamfang (talk) 20:09, 28 December 2008 (UTC)
- With the price of copper these days aluminium has the highest conductivity for the cost, I am fairly sure it also has higher conductance than copper for a given mass too. Noodle snacks (talk) 11:05, 30 December 2008 (UTC)
- I seem to remember hearing that silver is the best conductor among wires of the same thickness, copper is best with respect to weight, and aluminum is next best either way. —Tamfang (talk) 20:09, 28 December 2008 (UTC)
Planet destroying energy
[edit]How big would a nuclear blast need to be to destroy Earth? To actually obliterate it into chunks? I stumbled upon this page [1] which shows the size of the Death Star in relation to the moon. I can't imagine something so small blowing up an Earth-like planet that's 60x bigger than the moon. Granted, it's science fiction. But what quantity of energy would the Death Star need to generate to blow up a planet? Is that even in the realm of fusion or matter/anti-matter reactions? --70.167.58.6 (talk) 05:37, 22 December 2008 (UTC)
- You can't quite compare an explosion with an Impact force. The two behave differently as you can see from those pages. 76.97.245.5 (talk) 07:10, 22 December 2008 (UTC)
- You need to overcome gravitational binding energy. The value for Earth is a bit larger than the number given in the article because Earth is not of uniform density; it's about 2.4*1032 J. That's the energy equivalent of 2.7*1015 kg. If the chunks are as small as they appear in Star Wars, the gravitational binding energy of these chunks is negligible compared to the 2.4*1032 J, so you'll need approximately that amount of energy. Icek (talk) 08:50, 22 December 2008 (UTC)
- For comparison, the theoretical maximum yield of the Tsar Bomba, the largest fusion weapon ever tested, was 100 Mt or 4.2x1017 J (the actual test bomb was limited to a yield of 50 Mt). So, even if all of this energy could be utilised, you would need 5x1014 (i.e. 500 trillion) such bombs to reduce the Earth to small chunks. Gandalf61 (talk) 09:17, 22 December 2008 (UTC)
- You'd also have to give those chunks enough speed to exceed their mutual escape velocity - or else they'd all come back together in a gigantic collision and form a new planet ("NEW!! Earth 2.0TM - now with fewer annoyingly destructive lifeforms!"). SteveBaker (talk) 14:04, 22 December 2008 (UTC)
- Hmmm.... what do you mean? You can do this if you have 2.4*1032 J at your disposal - at least in principle. Icek (talk) 14:19, 22 December 2008 (UTC)
- A pretty good website about it can be found at [2], and some technical data here: [3] --86.125.162.148 (talk) 18:47, 22 December 2008 (UTC)
- The energy "to exceed their mutual escape velocity" is what gravitational binding energy means. —Tamfang (talk) 20:14, 28 December 2008 (UTC)
Gravity hole
[edit]Could there theoretically be an equivalent for black holes when we are talking about gravity and gravitons ? 69.157.229.14 (talk) 05:40, 22 December 2008 (UTC)
- What type of "equivalent" for a black hole are you trying to define? AFA our article states a black hole is a phenomenon related to gravity. Are you trying to have a location not influenced by gravity? (I think that would be impossible, the best you can hope for is offsetting forces e.g. with a magnetic field.[4]) Gravitons are (hypothetical) particles that "carry" gravitational forces, sort of like electrons carry electrical charge. or Are you thinking of supersymmetry, creating an "anti-black hole" with Gravitinos?? P.S. I put hypothetical in brackets so that we don't end up with another one of those "it's only a theory discussions". No one's found one yet, but there are a lot of clues saying there should be one, unless s.o. is going to come up with a better explanation. 76.97.245.5 (talk) 06:56, 22 December 2008 (UTC)
- I guess you're asking about a region of space from which gravitons can't escape. That would be a black hole. Nothing can escape across the event horizon of a black hole, including gravity. The field of a black hole is a so-called "fossil field" left over from the collapse. It doesn't come from inside the hole. -- BenRG (talk) 08:08, 22 December 2008 (UTC)
- I don't think that's right. A black hole's gravitational attraction to other objects does increase with every object it swallows, doesn't it ? Therefore, those theoretical gravitons must escape. Perhaps this is a flaw in graviton theory itself, and visualizing gravity as a deformation in space-time is more accurate. StuRat (talk) 18:43, 22 December 2008 (UTC)
- Yes - it's much easier to think of gravity as space-time deformation. But (to pre-empt Jayron32 who is just ITCHING to say this) "Gravity is gravity, however, and that it sometimes behaves like a space-time deformation and sometimes like a particle is not gravities problem. Its ours."
- But it seems entirely unreasonable to me that a graviton is affected by gravity. That doesn't sound right. The (hypothetical) graviton is the force-carrier particle for a gravitational field - just like the photon is for an electromagnetic field. It's what communicates the 'message': "There is a damned great black hole over there - and therefore you should be accelerating towards it!".
- Photons don't get bent around by electric fields - so why would we expect gravitons to be affected by a gravitational field? If something in a large chunk of matter is emitting gravitons - and if those gravitons are affected by that gravity - then you'd need a second graviton to carry the message to the first one...then what communicates the message to the second graviton?!? That's just got to be wrong.
- So I think BenRG is incorrect - if gravitons exist - they can escape a black hole because they aren't affected by gravity.
- The business of the 'fossil field' is to do with the relativistic issues of matter entering the black hole being 'frozen' at it's surface by time dilation.
- SteveBaker (talk) 21:19, 22 December 2008 (UTC)
- I have lots of other problems with gravitons, or any gauge bosons, really. They all have in common that a seemingly infinite number of undetectable particles be constantly bouncing between every pair of elemental particles in the universe. StuRat (talk) 21:51, 22 December 2008 (UTC)
- StuRat: Massive particles that fall into a black hole have gravitational fields of their own. When they get close to the event horizon, you feel the combination of the two fields, which is similar to the field of a uniform (no hair) black hole of the combined mass. Over time the inhomogeneities in the field radiate away and you end up with a larger uniform field.
- SteveBaker: Everything gravitates, including the gravitational field. That's one way of understanding why GR is nonlinear: the field interacts with itself. It's classical electromagnetism that's the oddball here—all of the forces in the Standard Model are nonlinear, including electromagnetism, though the nonlinearity there is small. The strong force is extremely nonlinear: the gluons have color charge and the coupling constant is very large.
- StuRat, and everyone really: Feynman diagrams are ridiculously oversold in popular books, to the point that a lot of people seem to think that quantum field theory is about virtual particle exchange and you can't have a quantum field theory without virtual particles. That's not how it works at all. Quantum field theory is about fields. Virtual particles and Feynman diagrams are a kind of series expansion for the underlying field theory, similar to a Taylor series. Taylor series are great when they converge quickly, not so great when they converge slowly, and useless outside the radius of convergence where they don't converge at all. The perturbation theory in Feynman diagrams is the same way. You can't "see" the whole field theory through Feynman diagrams, and what you do see is arguably not conducive to a good understanding of the full theory. If you do gravity with Feynman diagrams you have to choose a fixed background and perturb around that, which means you lose the background-free character of GR, which is really the most interesting thing about it. The Standard Model forces also have some of that background-free character—that's what the "gauge" in "gauge boson" means—and that's lost when you think of them in terms of Feynman diagrams. So forget about virtual bosons. "Virtual graviton exchange" is at best just another term for "gravitational attraction", and at worst it's a strictly less general notion. When I see questions about virtual gravitons I usually try to answer as though they were about fields, as I did above. -- BenRG (talk) 08:18, 23 December 2008 (UTC)
Thats an interesting point. So is there still a black hole inside?Trevor Loughlin (talk) 14:33, 22 December 2008 (UTC)
Speed of light in another universe
[edit]Could the laws of physics be different in another universe, and if so, could the speed of light be different as well? I heard somewhere that the laws of physics are mostly dictated by the nature of the particle that make up our universe. While this sound plausible, I'm sure it's far from fact. If it were true, could an alternate universe composed by alternative particles making what we would call exotic matter (but to them would be ordinary) then have different rules on how that matter acts? Tiailds (talk) 07:44, 22 December 2008 (UTC). I have shown that the speed of light in another universe is related to the mass of that universe. J Forrest see http://mayennegite.chez-alice.fr/Universes/.
- Can't quite see why you shouldn't be able to have a different universe with a different speed of light (in a vacuum!). The crux of the matter is that if you change one thing you have to see what else is affected. You can't just say "in this universe apples fall up". You would then also have to describe all the related issues e.g. how apple trees grow, what makes apple, falling up different from pears, falling down. Since it has taken many generations of physicists to sort out how things are connected in this universe, and we still haven't figured it all out to the last detail, I'd assume you'd have to live with some approximation of how a universe functions where light traverses a vacuum at a different speed, because it would take too long to define everything. 76.97.245.5 (talk) 08:28, 22 December 2008 (UTC)
Simply that the speed of light is determined by the space it is moving through, determined by the total effect object masses and gravitational effects in our universe. These determine the spatial geometry through which light moves so another (parallel) universe for example may have a larger total mass than ours which should change the spatial geometry, hence the speed of light. J Forrest Dec 2010
- By definition, we cannot know the laws of physics in another universe. Icek (talk) 08:38, 22 December 2008 (UTC)
- It's certainly true that we can't know. Indeed we can't even known that there ARE other universes (although it would certainly explain a whole lot if there were). There are some things like the speed of light or the charge on the electron that I think most physicists would be happy to accept could be different because we have no reason to know why they have the exact values they have in our universe (although the strong anthropic principle is some sort of a reason). But there are other things - more in the realms of mathematics - that I think we'd be unwilling to agree could possibly be different - the value of PI for example. It's fairly easy to imagine a universe where light travelled 0.000001% faster than it does in ours - but it's impossible to imagine a universe in which PI differs AT ALL from the value in ours!
- Somewhere between those limits we have physics/math things like dimensional analysis that say things like that 'velocity = frequency * wavelength' has to be true because velocity is measured in meters-per-second, frequency in 1/seconds and wavelength in meters - so for the dimensions of the equation to balance, it pretty much has to be that. A universe in which (say) velocity equals frequency SQUARED times wavelength would be seriously screwy! So I think we could be reasonably comfortable saying that some of the equations we're happy with in examining our universe would be fundamental enough to hold in other universes...but others, less so.
- If we're happy to entertain things like different values for the speed of light - then we would CERTAINLY expect matter to behave radically differently - it doesn't take much of a change in the charge on the electron to make all atomic matter impossible and reduce the universe into a bunch of black holes with nothing else present but vacuum. Matter with bizarre behavior (and therefore 'exotic' by our standards) would certainly be expected if any of the fundamental constants were off by a relatively small amount. So unless there is some deep underlying reason why the speed of light, the charge on the electron, the universal gravitational constant and the plank length are what they are - then they could be different - and all sorts of wierdness would result.
- But other universes might be different in other ways too - more or fewer spatial dimensions certainly seems like a reasonable possibility - and that would result in things being so seriously out-of-whack that it would be almost impossible to imagine the consequences. If string theory is right and our universe has 26 dimensions (or however many it is that string theorists are predicting this week!) - of which 23 are 'tightly coiled up' or 'small' and therefore impossible for us to detect - then who is to say that with different fundamental constants (like the speed of light) that they wouldn't have 17 'small' dimensions and 9 normal dimensions. Right there - we'd have a universe that would be hard to imagine and impossible to visualise.
- Indeed, if a universe would have 2 or 4 (ordinary) spatial dimensions and by analogy with the universe would have an attractive gravitational force proportional to 1/r or 1/r3, respectively, then planetary orbits wouldn't be stable - the only closed orbits would be circular (and I think this is true for any dimension other than 3; but if we look closely orbits aren't closed in the real universe either, even in the 2-body case - in General Relativity there is perihelion precession, and, even worse, gravitational radiation). Icek (talk) 14:29, 22 December 2008 (UTC)
- This is kind of off-topic, but in 2+1 dimensional general relativity there are no closed orbits, even unstable, even approximately. In fact, test particles do not accelerate toward massive objects in 2+1 dimensional general relativity. It's very different from 2-dimensional Newtonian gravity. -- BenRG (talk) 22:17, 22 December 2008 (UTC)
- It's hard to say what it means to "change the speed of light". Units like meters and seconds are based on human beings—a meter is about the size of a person and a second is about how long it takes to have a thought. If the speed of light were twice as fast, then we'd think twice as fast, so our second would be half as long, so the speed of light in human-based units would be the same. In some sense 299,792,458 m/s is not a physical constant at all, it's a biological constant, a measure of how much low-level signaling in the brain is needed for conscious thought. What about the speed of light in "natural units"? Well, in the usual "natural units", Planck units, the speed of light is 1 and can't be changed because it's how those units are defined in the first place. So our attempt to change the speed of light failed to change its value in natural units or in human units—in what sense did it change at all? People sometimes say that you can avoid those problems by only talking about unitless constants, but that's not really true either. For example, the proton/electron mass ratio (about 1836.15267) is a unitless constant, but it's not clear what it means to change it in isolation. The problem is the arbitrariness of coordinates. The Standard Model of particle physics has about 26 continuous parameters, but not any particular 26, in the same way that space has three dimensions, but not any particular three. You can't change your x coordinate unless you know how the x coordinate is defined, and you can't change your x coordinate "without changing anything else" unless you know what the other coordinates are too. Nonetheless, you can choose a different point in the 26-dimensional parameter space and get a description of a different universe. It's just hard to say exactly what you've changed.
- Most if not all of the continuous parameters in the Standard Model can be interpreted as properties of particles, so in that sense "the laws of physics are mostly dictated by the nature of the particles". In fact (as John Baez mentions in the above-linked web page) most of the constants can be interpreted as properties of the Higgs boson, which is what makes it so interesting. -- BenRG (talk) 22:17, 22 December 2008 (UTC)
- I disagree. I understand what you're saying: I suppose you can say that if all of the fundamental constants that relate to time and distance were scaled by the exact same amount - and all of the ones that relate to mass by another...and so on...then the 'absolute' values for these constants could change and you wouldn't notice a difference...and unless you could somehow transport a stopwatch, a tape measure and some kilogram test masses from our universe to the other - there would be no way to know that there even was a difference. So in that sense, two universes could have different speeds of light - and so long as all of the other time and distance values were scaled proportionately - you'd never know.
- But if the speed of light was different - yet none of the other constants containing time and distance were ALSO different - then things would behave differently in fundamental and noticable ways. Restating things in Plank units doesn't change that fact. In the article you linked, the masses of the quarks (in Plank units) are now fundamental constants. In that scheme, if the RATIO of the masses of the charm and strange quarks were different than in our universe but the ratio of the up and strange quarks were exactly the same - then we'd have a different universe no matter what units or scaling you apply to it. Unless we can find a fundamental reason WHY the quarks have those particular masses - so we can eliminate all of the quark-masses from the list of constants and relate them all to something more fundamental - then they can (in principle) be different in other universes. But worse still - many of the constants are dimensionless. Change one of those and the universe is different no matter what because you can't compensate for that change.
- But in the end we don't (and can't) know...it's perfectly possible for another universe to have no analogs of matter or energy or time or space or anything else - and for our universe to lack their ubiquitous snark, blarth, pling (and anti-pling) upon which all of everything is based on their side of reality. SteveBaker (talk) 14:21, 23 December 2008 (UTC)
I quite agree that other universes could probably have an infinite number of other dimensions, hence different constants. However, my theory is only concerned about a parallel universe which influences ours which has mass and similar dimensions to ours which explains a variety of observations such as dark matter and energy, etc. The double-slit photon experiment also supports this idea that two (or more) universes can exist side-by-side and influence each other at a material and energy level and that the presence of a parallel universe is also likely to be influencing our universe at the atomic level. I have used this experiment to show that our immediate merged bu tsimilar parallel universe is only 16ms out of phase with ours which explains why we can detect its mass (dark matter) but cannot 'see' it because the light from it is out of phase with our detection methods. For this to work the Universes will need to be merged and nearly in-phase, hence sharing dimensions, mass and energy at spatial and atomic levels. Probability dictates that other parallel universes may influence ours but will be too out of phase to influence us significantly. J Forrest Dec 2010
Particle-wave separation/fusion
[edit]In light, can the particle ever disappear and leave only the wave, only to return a few instants later? 69.157.229.14 (talk) 08:16, 22 December 2008 (UTC)
- No, because that's complete nonsense. Light doesn't have separate wave and particle parts. Algebraist 08:35, 22 December 2008 (UTC)
- Have a look at Wave–particle duality.76.97.245.5 (talk) 08:39, 22 December 2008 (UTC)
- Light is neither a wave NOR a particle - but it behaves a bit like both of them - sometimes (eg in diffraction) it seems more like a wave and in other times (eg in the photoelectric effect) it seems more like a particle. But these are just ways to describe it's behavior in more or less familiar terms. Light (or more properly 'electromagnetic radiation') is quite different from other kinds of 'stuff' in the universe. So you can't separate out the wave and the particle behaviors because they aren't separate - they are just ways of thinking about something that lies beyond our normal day-to-day human experience. As Jayron32 is fond of telling us "Light is light, however, and that it sometimes behaves like a wave and sometimes like a particle is not lights problem. Its ours." - which sums it up rather eloquently. SteveBaker (talk) 13:09, 22 December 2008 (UTC)
- Another way to say it is that people have made up the concepts of waves and particles. They both happen to have some properties of light. — DanielLC 17:59, 22 December 2008 (UTC)
- Did I just get quoted there (ego swells)... Thanks Steve! I have nothing further to add, since SteveBaker already said what I was going to say!. --Jayron32.talk.contribs 20:19, 22 December 2008 (UTC)
- Don't get too excited - we're all bored with that one. Now you've got to find something cogent and witty to say about the lipid layer in the eye. :-) SteveBaker (talk) 20:45, 22 December 2008 (UTC)
- See the lipid layer is the lipid layer, however, and that it sometimes behaves... oh fuggit. It doesn't work as well there... --Jayron32.talk.contribs 14:20, 23 December 2008 (UTC)
- I like to use the term "wavicle" to describe light: poke it one way and it looks like a wave, poke it another way and it looks like a particle. --Carnildo (talk) 02:23, 24 December 2008 (UTC)
Storing solar lights
[edit]I got some solar x-mas lights this year. What is the best way to store them, so that they will work again next x-mas? Should I take out the rechargeable batteries? If yes, what's the best way to store them? Should I recharge them at regular intervals during the year? Should I let them drain completely before I put them away? Would it be better to run the lights in the back yard all year round than storing them till next x-mas? Other ideas?76.97.245.5 (talk) 08:48, 22 December 2008 (UTC)
- It depends on the kind of battery, but it's usually better to drain them "completely" (see memory effect, but also see "deep discharge" there) and then recharge them before you store them in order to prevent deep discharge. As for the lamps, if they are incandescent lamps they wear out quickly if you turn them on and off often. Just store them. Icek (talk) 12:17, 22 December 2008 (UTC)
- Thanks a lot for the info and the link. The lights are led, but I guess their lifespan doesn't improve with use either. I'll just run the batteries though my charger (that takes care if draining them, too) and then put everything away. 76.97.245.5 (talk) 12:50, 22 December 2008 (UTC)
- LED's last an awful long time and can take a lot of abuse - there probably isn't much you can do to hurt them. I would keep them someplace dark and dry (more for the sake of the wiring and connections than for the LED's themselves) - but otherwise no special care is needed. The batteries (as Icek said) should probably be allowed to completely discharge - ie turn the lights on - put the solar panel someplace dark until the lights go completely off then store them in the dark so it doesn't recharge! The only kinds of rechargeable battery that don't like to be stored in a discharged state are lead/acid batteries (like car batteries) that should be kept fully charged at all times - but they don't use lead/acid for things like solar-powered lights so that's not a problem here. When you take them out next year - wipe the cover that's over the solar panel to make sure it's clean and you're good to go. SteveBaker (talk) 12:59, 22 December 2008 (UTC)
- Well, Li-ion batteries don't like to be stored discharged, either. I think 40% is the optimal charge for long-term storage, at least for Li-ion polymer batteries. -- Aeluwas (talk) 13:55, 22 December 2008 (UTC)
- More like 75% for all lithium batteries131.172.99.15 (talk) 14:01, 23 December 2008 (UTC)snaxalotl
- LED's last an awful long time and can take a lot of abuse - there probably isn't much you can do to hurt them. I would keep them someplace dark and dry (more for the sake of the wiring and connections than for the LED's themselves) - but otherwise no special care is needed. The batteries (as Icek said) should probably be allowed to completely discharge - ie turn the lights on - put the solar panel someplace dark until the lights go completely off then store them in the dark so it doesn't recharge! The only kinds of rechargeable battery that don't like to be stored in a discharged state are lead/acid batteries (like car batteries) that should be kept fully charged at all times - but they don't use lead/acid for things like solar-powered lights so that's not a problem here. When you take them out next year - wipe the cover that's over the solar panel to make sure it's clean and you're good to go. SteveBaker (talk) 12:59, 22 December 2008 (UTC)
Earth's rotation
[edit]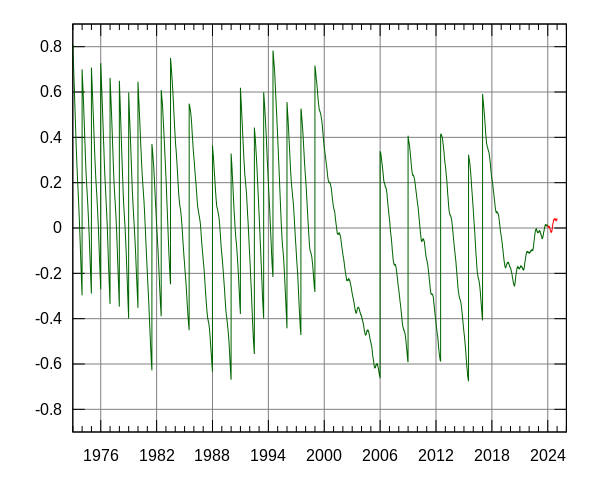
Looking at the image, Earth apparently spins up every year approximately at the time of Northern Summer. Is this because of snowfall in Antarctic winter which lowers the sea level a bit? (Earth's moment of inertia is about 8*1037 kg m2) it would mean that sea levels change seasonally by maybe 0.2 m (I haven't done a proper calculation) which sounds like too much. Is there another important cause of the seasonal variation? And why was Earth rotating so slow in recent years (no leap second in the years 2000 - 2005)? Icek (talk) 10:30, 22 December 2008 (UTC)
- This term is dubbed secular acceleration, which is the phenomenon of earth slowing down every year. ♫Deathgleaner 00:48, 27 December 2008 (UTC)
- Someone on sci.physics said that the leaves on trees contribute to the effect (most trees are in the Northern Hemisphere), but the effect of that would be the opposite of what you noticed... -- Army1987 – Deeds, not words. 10:52, 22 December 2008 (UTC)
- Indeed, the faster the Earth spins the steepest the descent of the curve is, so, to me, it seems that it's faster in winter, as the above would predict. -- Army1987 – Deeds, not words. 10:58, 22 December 2008 (UTC)
- No, the slower the Earth spins the steeper the descent of the curve is: Then leap seconds have to be more frequently, as can be easily seen from the diagram. A leap second means that the Earth hasn't yet spun as far as the 86400 second day would predict and we have to wait an extra second for Earth.
- As for the leaves on trees: If the average height of the leaf above ground is h = 20 m, and there is A = 40 million km2 more deciduous forest on the Northern Hemisphere than on the Southern Hemisphere (an gross overestimation I think), and the effective (not quite the average) lattitude is θ = 35°, and there is one tree per At = 10 m2, and there is ml = 200 kg leaves per tree, then the change in moment of inertia is:
- ΔI = 2 * r(θ) * h*cos(θ) * A/At * ml = 1.37*1023 kg m2
- where r(θ) = REarth*cos(θ) is the distance from Earth's axis at the effective lattitude. This is a relative change of moment of inertia - and thus of angular velocity - of 1.7*10-15. I think I certainly didn't underestimate with my choice of the parameters. That relative change would mean a change in slope of the curve equivalent to an extra leap second (or a missing leap second) once every 18.5 million years - and that is clearly way less than what we see in the diagram! Icek (talk) 11:53, 22 December 2008 (UTC)
- By the way, the relative change is also at the current limits of accuracy in measuring time. Icek (talk) 11:55, 22 December 2008 (UTC)
- No expertise in this but I would expect the earth to be slower in recent years with global warming because the water would be spread out rather than at the poles. The spin up during summer may be because the water goes into the ocean instead of staying on the land. Dmcq (talk) 13:12, 22 December 2008 (UTC)
- That's what puzzles me - it's that Earth rotated faster in recent years. How do you think water going into the ocean instead of staying on the land causes Earth to spin up? If some amount of mass is located at a certain lattitude θ and then gets spread out over the whole surface of Earth (yes, I'm ignoring the distribution of water and land by lattitude, I could be substantially more accurate by a detailed calculation), it will decrease Earth's moment of inertia only if it was at a lattitude smaller than arccos(sqrt(2/3)) = 35.26°, and else it will increase Earth's moment of inertia (and thus slow down Earth's rotation). Icek (talk) 14:39, 22 December 2008 (UTC)
- The answer (for the seasonal variation) seems to be the exchange of angular momentum between the atmosphere and the the lower part of Earth [5]. Icek (talk) 15:16, 22 December 2008 (UTC)
- The atmosphere's mass is about 5*1018 kg, so its angular momentum is about M*R2*2/3 = 1.35*1032 kg m2. The effect is a relative change of maybe 10-8; that means an average angular velocity of the atmosphere of 0.006 times Earth's angular velocity - which is just 2.7 m/s at the equator. Of course not the whole atmosphere is moving, but the speed will not be that much lower closer to the poles as my calculation assumes (i. e. not like a rigid body), and it seems realistic. Icek (talk) 15:28, 22 December 2008 (UTC)
- I think we are grasping at straws here. During northern hemisphere summer, the earth is at its aphelion, its farthest point from the sun. Being farther from the sun, the earth will experience less tidal drag from the sun, thus speeding up its rotation slightly. All the bullshit about this being due to changes in weight distribution due to increased snowfall at the south pole, or leaves falling off of trees, or anything like that seems like some pretty wild stuff. None of those is going to make an effect like this, I am pretty sure its just that, since we are farther away from the sun, we spin a little faster... --Jayron32.talk.contribs 20:16, 22 December 2008 (UTC)
- Yep - I agree. These other things seem vastly too small to have a significant effect - tidal forces from the sun account for annual variations - the moon's gradual slowing down in orbit and the drag due to turbulence in the core can account for longer term effects. SteveBaker (talk) 20:41, 22 December 2008 (UTC)
- Here's an article that doesn't need subscribing A New Spin on Earth's Rotation. I must admit to being surprised the atmosphere has such a large effect. Dmcq (talk) 22:24, 22 December 2008 (UTC)
I have to disagree with Jayron32 - tidal forces are proportional to m/r3 (with m being the mass of the body causing the tidal forces and r being the distance from this body), so the relative strength of Moon's and Sun's tidal forces can be easily calculated. The Sun's tidal forces on Earth are a bit less than 1/2 that of the Moon, and the difference between the Sun's tidal forces at aphelion (152.1 Gm) and perihelion (147.1 Gm) is a bit less than 1/20 that of the Moon's total tidal forces. That means that the seasonal change due to this effect should be less than the long term slowing due to tidal forces. But from the diagram alone one can see that the total seasonal variation is far larger. Icek (talk) 05:13, 23 December 2008 (UTC)
- I disagree. You're comparing long term slowing due to the gradual slowing down of the moon in it's orbit to seasonal slowing - you should be comparing monthly effects of the moon to the annual effect of the sun. The moon raises tides comprising around 100,000 cubic kilometers of water - that dwarfs all of the other effects described (leaves, etc) - and even 1/20th of that (seasonally) is an immense variation. We'd probably see even greater lunar-monthly day-duration changes were it not for the dampening effect of the liquid core and the large inertia of the earth...but an effect that happens on an annual basis is long-lived enough to produce a measurable effect. SteveBaker (talk) 13:54, 23 December 2008 (UTC)
- I don't think you are correct here: The tides do not directly change the effective moment of inertia by creating bulges of water, because these bulges don't rotate with Earth. So the change in rotation rate is by friction only, and there shouldn't be an increase in rotation rate when the tidal forces become weaker, but the diagram clearly shows an increase.
- Regarding the atmospheric effect, note that this is not a redistribution of mass as in the cases of falling leaves and melting polar glaciers; it is that 2 parts (there are actually more than 2, but you get the idea) of Earth have different rotation rates, and we're measuring only one part. The atmosphere spins up in Northern winter and takes angular momentum from the rest of Earth beneath it. Icek (talk) 14:59, 23 December 2008 (UTC)
- But there's just not enough atmosphere to have that effect! The mass of the atmosphere relative to the solid part of the earth is just not enough to cause such rather large changes in momentum. One second per year seems rather high for such effects, even if the entire atmosphere shifted to between the poles over the course of a year, we probably wouldn't see such effects. Yes, there are effects slowing the earth down and speeding it up due to redistribution of mass due to "tidal bulges" and the like, but even if the earth was a perfectly rigid ball, there would be a drag effect caused by the force of gravity, which is pulling against the spin of the earth, and I would think THAT effect is greater than any caused by such esoteric and tiny factors as Northern Hemisphere weather... --Jayron32.talk.contribs 18:21, 23 December 2008 (UTC)
- Atmosphere moment of inertia (~2/3 M R^2): ~1.3 ×1032 m2 kg. Moment of inertia of solid Earth (~2/5 M R^2): 8 ×1037 m2 kg. So the ratio of the atmosphere to the solid earth moment of inertia is 1.6 parts per million. A variation of one second per year is 1 part in 32 million. In other words, you only need a 5% change in the angular momentum of the atmosphere to add 1 second to the angular momentum of the solid Earth (or vice versa). Dragons flight (talk) 18:52, 23 December 2008 (UTC)
- Jayron32, how do you think that "a drag effect caused by the force of gravity..." will change the angular momentum of a rigid ball? How do you get a torque? Icek (talk) 21:08, 23 December 2008 (UTC)
New image and new headline
[edit]Because the annual variations are hard to see in the original image, I plotted the same data over the course of a year. The thin green lines connect consecutive days; the almost horizontal lines are distracting, they are the connections between Dec 31 and Jan 1 of the next year. The big spike occurs on 5/19/1976, it is obviously an error because the next day ∆t is given as 0. — Sebastian 22:02, 23 December 2008 (UTC)
The next highest two peaks occur on Jan 5 1833 and at the beginning of Feb 1983. — Sebastian 22:15, 23 December 2008 (UTC)
- Thanks for the image (for anyone else interested in the data: link, format). What you plotted is the derivative of the data in the original image (or isn't it?) - so here a positive slope means that Earth's rotational period is increasing and a negative slope means that Earth is spinning up, while in the original image, a constant slope meant that Earth's rotational period stays constant. One can see that there are monthly variations: When looking closer at the data it seems like there are local minima of the length of day both at Moon's apogee and perigee (the last perigee was on or around December 13, according to Heavens-Above), and the length of day is larger at the perigee (i. e. shortly before the Moon reaches perigee Earth spins up, after perigee it slows down again and reaches a minimum of angular velocity before apogee; then it spins up again in order to reach its largest angular velocity at apogee, and then again slows down to another minimum before perigee and so on). I don't know exactly why this is so, but it's a more subtle effect than what SteveBaker and Jayron32 seem to be thinking. Icek (talk) 09:04, 24 December 2008 (UTC)
- Yes, it's the derivative. To be exact, it's the negative of the derivative of UT1-UTC, (or, if you like, the derivative of UTC-UT1) because I wanted the value to be predominantly positive. I'm now aware that that may not have been a good idea, and apologize for any confusion this may have caused. I should also mention that the "months" are actually exactly 1/12 years; I compensated for different month lengths. — Sebastian 04:14, 26 December 2008 (UTC)
- I think plotting Earth's rotational period (or rather the difference between Earth's rotational period and 24 hours) is actually better than the original image for the purpose of this discussion. Even the second derivative of the original image wouldn't be all that bad - then one could conclude that some feature is not caused by friction if the curve is below zero. Icek (talk) 16:16, 26 December 2008 (UTC)
- I'm now inclined to think that it's really mostly the eccentricity of Earth's orbit: Looking at the data, there are local minima of length of day at both aphelion and perihelion fairly regularly (well, often the perihelion minimum is not quite visible). But why? It seems like changes in effective angular momentum are dominated by the absolute value of the rate of deformation in some way - I don't understand how this works. Icek (talk) 09:30, 24 December 2008 (UTC)
the nearest galaxy
[edit]hello, i was wondering, what is the nearest galaxy to ours? —Preceding unsigned comment added by 92.13.130.144 (talk) 18:35, 22 December 2008 (UTC)
- The Milky Way (the galaxy which we are in) is part of the Local Group, a cluster of about 35 galaxies (that article, and ones it links, has a lot of good information about nearby galaxies). The nearest spiral galaxy is the Andromeda galaxy (also in the local group). The nearest galaxy of any shape is a little harder to describe. The nearest one of any significant size is the Large Magellanic Cloud. There are dwarf galaxies which are closer, such as the Canis Major Dwarf Galaxy, which is only 42,000 light year from the galactic center, or 25,000 light years from earth. According to the article it "is now thought to be the closest neighbouring galaxy to our location in the Milky Way". However, it was only discovered in 2003 (the Sagittarius Dwarf Elliptical Galaxy held the previous record), so there may be a possibility that an as-yet-undiscovered galaxy is closer still. -- 128.104.112.113 (talk) 18:48, 22 December 2008 (UTC)
Acceleration of particles in a secluded system
[edit]I read that space-time travel could be reached by means of acceleration of particles in a secluded system. What would this mean concretely ? 69.157.229.14 (talk) 20:54, 22 December 2008 (UTC)
- Er..."space-time travel"? I think we first need to ask what you mean by that. Travelling in space over the course of time is what we normal people call "moving". And it's impossible to achieve movement without some acceleration of particles...but if particles are accelerating then you have movement in space (and time is doing it's usual thing). It doesn't matter whether they are 'secluded' or not.
- If you actually mean just "time travel" - then no.
- I think you need to clarify what exactly it is you are talking about here. SteveBaker (talk) 21:28, 22 December 2008 (UTC)
I'm not sure, but it has something to do with this. [6][7] 69.157.229.14 (talk) 21:51, 22 December 2008 (UTC)
- The first link is a joke, the second is a video of a sculpture of a machine that doesn't work.... —Preceding unsigned comment added by Philc 0780 (talk • contribs) 22:20, 22 December 2008
- The second video claims to be of a time machine that travels into the future at a rate of 1 SPS (which one assumes means 'second per second') - which is an old and rather lame joke. However, taken at face value, it DOES work - it just doesn't do anything useful. SteveBaker (talk) 13:41, 23 December 2008 (UTC)
Tech Bump
[edit]Look at the palm of your right hand (if you are right handed). As you face it, I believe you may find a noticable bump in the bottom left corner. I'm convinced it's a souveneir from all the time that palm lies on a table as the hand grasps a mouse. For the palm of graphics developers, this bump is absolutely inflamed and even painful. Is there a name for this scar? Sappysap (talk) 23:36, 22 December 2008 (UTC)
- Please excuse my "original research" but, no bump here! -hydnjo talk 23:47, 22 December 2008 (UTC)
- The hamate bone is often palpable near the hypothenar eminence. This response is simply descriptive regarding the location you describe - the RefDesk is NOT a place to ask for medical advice. --Scray (talk) 00:10, 23 December 2008 (UTC)
- Sorry, where was the request for medical advice? WP:OR none found here. Edison (talk) 00:25, 23 December 2008 (UTC)
- Thank you, Edison. Sappysap (talk) 00:27, 23 December 2008 (UTC)
- I just felt the need to be explicit about what I was saying - that I was describing a structure at that location, as requested, but not explaining the "inflamed and even painful" bump described by the OP. I guess this came off wrong, since Sappysap thanked you. Sorry about any inferred negativism. --Scray (talk) 00:39, 23 December 2008 (UTC)
- Thank you, too, Scray. Sappysap (talk) 00:47, 23 December 2008 (UTC)
- Sorry, where was the request for medical advice? WP:OR none found here. Edison (talk) 00:25, 23 December 2008 (UTC)
- Have you looked at Callus? Irritation and chronic Inflammation might also hold interesting info for you. 76.97.245.5 (talk) 00:15, 23 December 2008 (UTC)
- I am right handed but I hold the mouse with my left, so the mouse bump should be left. Thinking about how the hand would be resting on the table it would be sustained by the flesh at the lower, inner side of the outer edge of the hand. This flesh would be slightly shifted upwards and there would be a bump. Now I'm anxious not to really look at my hands to avoid original research. 95.112.186.8 (talk) 07:53, 23 December 2008 (UTC)
- First I went to Repetitive strain injury which seemed to fulfil the condition in concept only, the best is Splints even though it's for a horse, it seems closer to what you mean. Take from it what you can, Julia Rossi (talk) 10:00, 23 December 2008 (UTC)
- That bump is the pisiform bone, not the hamate. It is a sesamoid bone, a bone that develops within a ligament/tendon. (Party trick: If you use your other hand to push the pisiform distally [i.e. from your wrist toward your pinky)], it will press on the branch of the ulnar nerve that supplies the palmaris brevis muscle and slightly wrinkle the skin on the side of the palm beyond the wrist.) To avoid resting the weight of your hand on it, I'd suggest using an armrest on your chair so that your forearm is bearing the weight. -- Flyguy649 talk 17:17, 27 December 2008 (UTC)
Secondary minerals?
[edit]I was trying to improve a "random" page (on Wulfenite) and came across the term "secondary mineral". Searching WP, I found many pages that use the term, but no wiki links for it. Even though I am no geologist, I can guess what this term means, but it seems as though there should be a WP page to cite, or maybe there is a more precise term. Note that I am NOT asking for a definition - I'm looking for an appropriate wiki-link. Thanks. --Scray (talk) 23:58, 22 December 2008 (UTC)
- Minerals mentions secondary oxidation under "Sulfate class". Maybe you, or s.o. else could add a paragraph, then everyone could link there and we wouldn't need a new page. 76.97.245.5 (talk) 00:25, 23 December 2008 (UTC)
- Thanks. That content (in Mineral) is fairly narrowly-focused, and I don't have the knowledge to make a broader statement defining secondary minerals. Anyone else? --Scray (talk) 00:41, 23 December 2008 (UTC)
- I referred this question to the Talk:Mineral page. --Scray (talk) 01:00, 23 December 2008 (UTC)
- Secondary minerals are those formed under a weathering or oxidizing environment at or near Earth's surface, most often used in discussing surface expressions of ore deposits (least that's where I've seen the term most). That said, perhaps the best place for clarification would be the weathering article ... still thinking. Vsmith (talk) 14:45, 23 December 2008 (UTC)
- For those interested, this was resolved very nicely by Vsmith! --Scray (talk) 03:38, 24 December 2008 (UTC)