History of the LED


The first Light-Emitting Diode (LED) was created in 1927 by Russian inventor Oleg Losev[1], and used silicon carbide as a semiconductor. However, electroluminescence as a phenomenon was discovered twenty years earlier by the English experimenter Henry Joseph Round of Marconi Labs, using the same crystal and a cat's-whisker detector.[2][3] Despite having distributed his report in Soviet, German and British scientific journals, Losev's LED found no practical use for several decades, partly due to the very inefficient light-producing properties the semiconductor used.[4][5]

In 1936, Georges Destriau observed that electroluminescence could be produced when zinc sulphide (ZnS) powder is suspended in an insulator and an alternating electrical field is applied to it. In his publications, Destriau often referred to luminescence as Losev-Light. Destriau worked in the laboratories of Madame Marie Curie, also an early pioneer in the field of luminescence with research on radium.[6][7]
Hungarian Zoltán Bay together with György Szigeti patenting a lighting device in Hungary in 1939 based on silicon carbide, with an option on boron carbide, that emitted white, yellowish white, or greenish white depending on impurities present.[8] Kurt Lehovec, Carl Accardo, and Edward Jamgochian explained these first LEDs in 1951 using an apparatus employing SiC crystals with a current source of a battery or a pulse generator and with a comparison to a variant, pure, crystal in 1953.[9][10]
Rubin Braunstein[11] of the Radio Corporation of America reported on infrared emission from gallium arsenide (GaAs) and other semiconductor alloys in 1955.[12] Braunstein observed infrared emission generated by simple diode structures using gallium antimonide (GaSb), GaAs, indium phosphide (InP), and silicon-germanium (SiGe) alloys at room temperature and at 77K. In 1957, Braunstein further demonstrated that the rudimentary devices could be used for non-radio communication across a short distance. As noted by Kroemer[13] Braunstein "…had set up a simple optical communications link: Music emerging from a record player was used via suitable electronics to modulate the forward current of a GaAs diode. The emitted light was detected by a PbS diode some distance away. This signal was fed into an audio amplifier and played back by a loudspeaker. Intercepting the beam stopped the music. We had a great deal of fun playing with this setup."

In September 1961, while working at Texas Instruments in Dallas, Texas, James R. Biard and Gary Pittman discovered near-infrared (900 nm) light emission from a tunnel diode they had constructed on a GaAs substrate.[14] By October 1961, they had demonstrated efficient light emission and signal coupling between a GaAs p-n junction light emitter and an electrically isolated semiconductor photodetector.[15] On August 8, 1962, Biard and Pittman filed a patent titled "Semiconductor Radiant Diode" based on their findings, which described a zinc-diffused p–n junction LED with a spaced cathode contact to allow for efficient emission of infrared light under forward bias.
After establishing the priority of their work based on engineering notebooks predating submissions from G.E. Labs, RCA Research Labs, IBM Research Labs, Bell Labs, and Lincoln Lab at MIT, the U.S. patent office issued the two inventors the patent for the GaAs infrared light-emitting diode (U.S. Patent US3293513), the first practical LED.[14] Immediately after filing the patent, Texas Instruments (TI) began a project to manufacture infrared diodes. In October 1962, TI announced the first commercial LED product (the SNX-100), which employed a pure GaAs crystal to emit an 890 nm light output.[14] In October 1963, TI announced the first commercial hemispherical LED, the SNX-110.[16]
In the 1960s, several laboratories focused on LEDs that would emit visible light. A particularly important device was demonstrated by Nick Holonyak on October 9, 1962, while he was working for General Electric in Syracuse, New York. The device used the semiconducting alloy gallium phosphide arsenide (GaAsP). It was the first semiconductor laser to emit visible light, albeit at low temperatures. At room temperature it still functioned as a red light-emitting diode. GaAsP was the basis for the first wave of commercial LEDs emitting visible light. It was mass produced by the Monsanto and Hewlett-Packard companies and used widely for displays in calculators and wrist watches.[17][18][19]

M. George Craford,[20] a former graduate student of Holonyak, invented the first yellow LED and improved the brightness of red and red-orange LEDs by a factor of ten in 1972.[21] In 1976, T. P. Pearsall designed the first high-brightness, high-efficiency LEDs for optical fiber telecommunications by inventing new semiconductor materials specifically adapted to optical fiber transmission wavelengths.[22]

Initial commercial development
[edit]
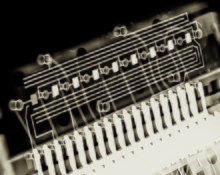
Until 1968, visible and infrared LEDs were extremely costly, on the order of US$200 per unit, and so had little practical use.[23] The first commercial visible-wavelength LEDs used GaAsP semiconductors and were commonly used as replacements for incandescent and neon indicator lamps, and in seven-segment displays, first in expensive equipment such as laboratory and electronics test equipment, then later in such appliances as calculators, TVs, radios, telephones, as well as watches.[24]
The Hewlett-Packard company (HP) was engaged in research and development (R&D) on practical LEDs between 1962 and 1968, by a research team under Howard C. Borden, Gerald P. Pighini at HP Associates and HP Labs.[25] During this time HP collaborated with Monsanto Company on developing the first usable LED products.[26] The first usable LED products were HP's LED display and Monsanto's LED indicator lamp, both launched in 1968.[26]
Monsanto was the first organization to mass-produce visible LEDs, using Gallium arsenide phosphide (GaAsP) in 1968 to produce red LEDs suitable for indicators.[23] Monsanto had previously offered to supply HP with GaAsP, but HP decided to grow its own GaAsP.[23] In February 1969, Hewlett-Packard introduced the HP Model 5082-7000 Numeric Indicator, the first LED device to use integrated circuit (integrated LED circuit) technology.[25] It was the first intelligent LED display, and was a revolution in digital display technology, replacing the Nixie tube and becoming the basis for later LED displays.[27]
In the 1970s, commercially successful LED devices at less than five cents each were produced by Fairchild Optoelectronics. These devices employed compound semiconductor chips fabricated with the planar process (developed by Jean Hoerni,[28][29] ). The combination of planar processing for chip fabrication and innovative packaging methods enabled the team at Fairchild led by optoelectronics pioneer Thomas Brandt to achieve the needed cost reductions.[30] LED producers have continued to use these methods as of about 2009.[31]
The early red LEDs were bright enough for use as indicators, as the light output was not enough to illuminate an area. Readouts in calculators were so small that plastic lenses were built over each digit to make them legible. Later, other colors became widely available and appeared in appliances and equipment.
Early LEDs were packaged in metal cases similar to those of transistors, with a glass window or lens to let the light out. Modern indicator LEDs are packed in transparent molded plastic cases, tubular or rectangular in shape, and often tinted to match the device color. Infrared devices may be dyed, to block visible light. More complex packages have been adapted for efficient heat dissipation in high-power LEDs. Surface-mounted LEDs further reduce the package size. LEDs intended for use with fiber optics cables may be provided with an optical connector.
Blue LED
[edit]The first blue-violet LED, using magnesium-doped gallium nitride was made at Stanford University in 1972 by Herb Maruska and Wally Rhines, doctoral students in materials science and engineering.[32][33] At the time Maruska was on leave from RCA Laboratories, where he collaborated with Jacques Pankove on related work. In 1971, the year after Maruska left for Stanford, his RCA colleagues Pankove and Ed Miller demonstrated the first blue electroluminescence from zinc-doped gallium nitride, though the subsequent device Pankove and Miller built, the first actual gallium nitride light-emitting diode, emitted green light.[34][35]
In 1974 the U.S. Patent Office awarded Maruska, Rhines, and Stanford professor David Stevenson a patent for their work in 1972 (U.S. Patent US3819974 A). Today, magnesium-doping of gallium nitride remains the basis for all commercial blue LEDs and laser diodes. In the early 1970s, these devices were too dim for practical use, and research into gallium nitride devices slowed.
In August 1989, Cree introduced the first commercially available blue LED, based on the indirect bandgap semiconductor, silicon carbide (SiC).[36] SiC LEDs had very low efficiency, no more than about 0.03%, but did emit in the blue portion of the visible light spectrum.[37][38]
In the late 1980s, key breakthroughs in GaN epitaxial growth and p-type doping[39] ushered in the modern era of GaN-based optoelectronic devices. Building upon this foundation, Theodore Moustakas at Boston University patented a method for producing high-brightness blue LEDs using a new two-step process in 1991.[40] In 2015, a US court ruled that three Taiwanese companies had infringed Moustakas's prior patent, and ordered them to pay licensing fees of not less than US$13 million.[41]
Two years later, in 1993, high-brightness blue LEDs were demonstrated by Shuji Nakamura of Nichia Corporation using a gallium nitride (GaN) growth process.[42][43][44] These LEDs had efficiencies of 10%.[45] In parallel, Isamu Akasaki and Hiroshi Amano of Nagoya University were working on developing the important GaN deposition on sapphire substrates and the demonstration of p-type doping of GaN. This new development revolutionized LED lighting, making high-power blue light sources practical, leading to the development of technologies like Blu-ray.[46][47]
Nakamura was awarded the 2006 Millennium Technology Prize for his invention.[48] Nakamura, Hiroshi Amano, and Isamu Akasaki were awarded the Nobel Prize in Physics in 2014 for "the invention of efficient blue light-emitting diodes, which has enabled bright and energy-saving white light sources."[49]
In 1995, Alberto Barbieri at the Cardiff University Laboratory (GB) investigated the efficiency and reliability of high-brightness LEDs and demonstrated a "transparent contact" LED using indium tin oxide (ITO) on (AlGaInP/GaAs).
In 2001[50] and 2002,[51] processes for growing gallium nitride (GaN) LEDs on silicon were successfully demonstrated. In January 2012, Osram demonstrated high-power InGaN LEDs grown on silicon substrates commercially,[52] and GaN-on-silicon LEDs are in production at Plessey Semiconductors. As of 2017, some manufacturers are using SiC as the substrate for LED production, but sapphire is more common, as it has the most similar properties to that of gallium nitride, reducing the need for patterning the sapphire wafer (patterned wafers are known as epi wafers). Samsung, the University of Cambridge, and Toshiba are performing research into GaN on Si LEDs.
Toshiba has stopped research, possibly due to low yields.[53][54][55][56][57][58][59] Some opt for epitaxy, which is difficult on silicon, while others, like the University of Cambridge, choose a multi-layer structure, in order to reduce (crystal) lattice mismatch and different thermal expansion ratios, to avoid cracking of the LED chip at high temperatures (e.g. during manufacturing), reduce heat generation and increase luminous efficiency. Sapphire substrate patterning can be carried out with nanoimprint lithography.[60][61][62][63][64][65][66]
GaN-on-Si is difficult but desirable since it takes advantage of existing semiconductor manufacturing infrastructure. It allows for the wafer-level packaging of LED dies resulting in extremely small LED packages.[67]
GaN is often deposited using metalorganic vapour-phase epitaxy (MOCVD),[68] and it also uses lift-off.
White LEDs and the illumination breakthrough
[edit]Even though white light can be created using individual red, green and blue LEDs, this results in poor color rendering, since only three narrow bands of wavelengths of light are being emitted. The attainment of high efficiency blue LEDs was quickly followed by the development of the first white LED. In this device a Y
3Al
5O
12:Ce (known as "YAG" or Ce:YAG phosphor) cerium-doped phosphor coating produces yellow light through fluorescence. The combination of that yellow with remaining blue light appears white to the eye. Using different phosphors produces green and red light through fluorescence. The resulting mixture of red, green and blue is perceived as white light, with improved color rendering compared to wavelengths from the blue LED/YAG phosphor combination.[69]

The first white LEDs were expensive and inefficient. The light output then increased exponentially. The latest research and development has been propagated by Japanese manufacturers such as Panasonic and Nichia, and by Korean and Chinese manufacturers such as Samsung, Solstice, Kingsun, Hoyol and others. This trend in increased output has been called Haitz's law after Roland Haitz.[70][71]
Light output and efficiency of blue and near-ultraviolet LEDs rose and the cost of reliable devices fell. This led to relatively high-power white-light LEDs for illumination, which are replacing incandescent and fluorescent lighting.[72][73]
Experimental white LEDs were demonstrated in 2014 to produce 303 lumens per watt of electricity (lm/W); some can last up to 100,000 hours.[74][75] Commercially available LEDs have an efficiency of up to 223 lm/W as of 2018.[76][77][78] A previous record of 135 lm/W was achieved by Nichia in 2010.[79] Compared to incandescent bulbs, this is a huge increase in electrical efficiency, and even though LEDs are more expensive to purchase, overall lifetime cost is significantly cheaper than that of incandescent bulbs.[80]
The LED chip is encapsulated inside a small, plastic, white mold[81][82] although sometimes an LED package can incorporate a reflector.[83] It can be encapsulated using resin (polyurethane-based), silicone,[84][85][86] or epoxy[87] containing (powdered) Cerium-doped YAG phosphor particles.[88] The viscosity of phosphor-silicon mixtures must be carefully controlled.[88] After application of a phosphor-silicon mixture on the LED using techniques such as jet dispensing,[89] and allowing the solvents to evaporate, the LEDs are often tested, and placed on tapes for SMT placement equipment for use in LED light bulb production. Some "remote phosphor" LED light bulbs use a single plastic cover with YAG phosphor for one[90] or several blue LEDs, instead of using phosphor coatings on single-chip white LEDs.[91] Ce:YAG phosphors and epoxy in LEDs[92] can degrade with use, and is more apparent with higher concentrations of Ce:YAG in phosphor-silicone mixtures, because the Ce:YAG decomposes with use.[93][94][95]
The output of LEDs can shift to yellow over time due to degradation of the silicone.[85] There are several variants of Ce:YAG, and manufacturers in many cases do not reveal the exact composition of their Ce:YAG offerings.[96] Several other phosphors are available for phosphor-converted LEDs to produce several colors such as red, which uses nitrosilicate phosphors,[97][98] and many other kinds of phosphor materials exist for LEDs such as phosphors based on oxides, oxynitrides, oxyhalides, halides, nitrides, sulfides, quantum dots, and inorganic-organic hybrid semiconductors. A single LED can have several phosphors at the same time.[89][99] Some LEDs use phosphors made of glass-ceramic or composite phosphor/glass materials.[100][101] Alternatively, the LED chips themselves can be coated with a thin coating of phosphor-containing material, called a conformal coating.[102][103]
The temperature of the phosphor during operation and how it is applied limits the size of an LED die. Wafer-level packaged white LEDs allow for extremely small LEDs.[67]
Polychromatic
[edit]In 2024, QPixel introduced as polychromatic LED that could replace the 3-subpixel model for digital displays. The technology uses a gallium nitride semiconductor that emits light of different frequencies modulated by voltage changes. A prototype display achieved a resolution of 6,800 PPI or 3k x 1.5k pixels.[104]
References
[edit]- ^ Losev, O. V. (1927). "Светящийся карборундовый детектор и детектирование с кристаллами" [Luminous carborundum detector and detection with crystals]. Телеграфия и Телефония без Проводов [Wireless Telegraphy and Telephony] (in Russian). 5 (44): 485–494. English translation: Losev, O. V. (November 1928). "Luminous carborundum detector and detection effect and oscillations with crystals". Philosophical Magazine. 7th series. 5 (39): 1024–1044. doi:10.1080/14786441108564683.
- ^ Round, H. J. (1907). "A note on carborundum". Electrical World. 19: 309.
- ^ Margolin J. "The Road to the Transistor". jmargolin.com.
- ^ Zheludev, N. (2007). "The life and times of the LED: a 100-year history" (PDF). Nature Photonics. 1 (4): 189–192. Bibcode:2007NaPho...1..189Z. doi:10.1038/nphoton.2007.34. Archived from the original (PDF) on May 11, 2011. Retrieved April 11, 2007.
- ^ Lee, Thomas H. (2004). The design of CMOS radio-frequency integrated circuits. Cambridge University Press. p. 20. ISBN 978-0-521-83539-8.
- ^ Destriau, G. (1936). "Recherches sur les scintillations des sulfures de zinc aux rayons". Journal de Chimie Physique. 33: 587–625. doi:10.1051/jcp/1936330587.
- ^ McGraw-Hill Concise Encyclopedia of Physics: electroluminescence. (n.d.) McGraw-Hill Concise Encyclopedia of Physics. (2002).
- ^ "Brief history of LEDs" (PDF).
- ^ Lehovec, K; Accardo, C. A; Jamgochian, E (1951). "Injected Light Emission of Silicon Carbide Crystals". Physical Review. 83 (3): 603–607. Bibcode:1951PhRv...83..603L. doi:10.1103/PhysRev.83.603. Archived from the original on December 11, 2014.
- ^ Lehovec, K; Accardo, C. A; Jamgochian, E (1953). "Injected Light Emission of Silicon Carbide Crystals". Physical Review. 89 (1): 20–25. Bibcode:1953PhRv...89...20L. doi:10.1103/PhysRev.89.20.
- ^ "Rubin Braunstein". UCLA. Archived from the original on March 11, 2011. Retrieved January 24, 2012.
- ^ Braunstein, Rubin (1955). "Radiative Transitions in Semiconductors". Physical Review. 99 (6): 1892–1893. Bibcode:1955PhRv...99.1892B. doi:10.1103/PhysRev.99.1892.
- ^ Kroemer, Herbert (Sep 16, 2013). "The Double-Heterostructure Concept: How It Got Started". Proceedings of the IEEE. 101 (10): 2183–2187. doi:10.1109/JPROC.2013.2274914. S2CID 2554978.
- ^ a b c Okon, Thomas M.; Biard, James R. (2015). "The First Practical LED" (PDF). EdisonTechCenter.org. Edison Tech Center. Retrieved 2016-02-02.
- ^ Matzen, W. T. ed. (March 1963) "Semiconductor Single-Crystal Circuit Development", Texas Instruments Inc., Contract No. AF33(616)-6600, Rept. No ASD-TDR-63-281.
- ^ Carr, W. N.; G. E. Pittman (November 1963). "One-watt GaAs p-n junction infrared source". Applied Physics Letters. 3 (10): 173–175. Bibcode:1963ApPhL...3..173C. doi:10.1063/1.1753837.
- ^ Kubetz, Rick (May 4, 2012). "Nick Holonyak, Jr., six decades in pursuit of light". University of Illinois. Archived from the original on July 10, 2020. Retrieved 2020-07-07.
- ^ Holonyak Nick; Bevacqua, S. F. (December 1962). "Coherent (Visible) Light Emission from Ga(As1−x Px) Junctions". Applied Physics Letters. 1 (4): 82. Bibcode:1962ApPhL...1...82H. doi:10.1063/1.1753706. Archived from the original on October 14, 2012.
- ^ Wolinsky, Howard (February 5, 2005). "U. of I.'s Holonyak out to take some of Edison's luster". Chicago Sun-Times. Archived from the original on March 28, 2006. Retrieved July 29, 2007.
- ^ Perry, T. S. (1995). "M. George Craford [biography]". IEEE Spectrum. 32 (2): 52–55. doi:10.1109/6.343989.
- ^ "Brief Biography — Holonyak, Craford, Dupuis" (PDF). Technology Administration. Archived from the original (PDF) on August 9, 2007. Retrieved May 30, 2007.
- ^ Pearsall, T. P.; Miller, B. I.; Capik, R. J.; Bachmann, K. J. (1976). "Efficient, Lattice-matched, Double Heterostructure LEDs at 1.1 mm from GaxIn1−xAsyP1−y by Liquid-phase Epitaxy". Appl. Phys. Lett. 28 (9): 499. Bibcode:1976ApPhL..28..499P. doi:10.1063/1.88831.
- ^ a b c Schubert, E. Fred (2003). "1". Light-Emitting Diodes. Cambridge University Press. ISBN 978-0-8194-3956-7.
- ^ Rostky, George (March 1997). "LEDs cast Monsanto in Unfamiliar Role". Electronic Engineering Times (944).
- ^ a b Borden, Howard C.; Pighini, Gerald P. (February 1969). "Solid-State Displays" (PDF). Hewlett-Packard Journal: 2–12. Archived (PDF) from the original on 2023-11-05.
- ^ a b Kramer, Bernhard (2003). Advances in Solid State Physics. Springer Science & Business Media. p. 40. ISBN 9783540401506.
- ^ "Hewlett-Packard 5082-7000". The Vintage Technology Association. Archived from the original on November 17, 2014. Retrieved 15 August 2019.
- ^ US 3025589, Hoerni, J. A., "Method of Manufacturing Semiconductor Devices", issued Mar 20, 1962
- ^ Patent number: 3025589 Retrieved May 17, 2013
- ^ Bausch, Jeffrey (December 2011). "The Long History of Light Emitting Diodes". Hearst Business Communications.
- ^ Park, S. -I.; Xiong, Y.; Kim, R. -H.; Elvikis, P.; Meitl, M.; Kim, D. -H.; Wu, J.; Yoon, J.; Yu, C. -J.; Liu, Z.; Huang, Y.; Hwang, K. -C.; Ferreira, P.; Li, X.; Choquette, K.; Rogers, J. A. (2009). "Printed Assemblies of Inorganic Light-Emitting Diodes for Deformable and Semitransparent Displays" (PDF). Science. 325 (5943): 977–981. Bibcode:2009Sci...325..977P. CiteSeerX 10.1.1.660.3338. doi:10.1126/science.1175690. OSTI 1876039. PMID 19696346. S2CID 8062948. Archived from the original (PDF) on October 24, 2015.
- ^ "Nobel Shocker: RCA Had the First Blue LED in 1972". IEEE Spectrum. October 9, 2014,
- ^ "Oregon tech CEO says Nobel Prize in Physics overlooks the actual inventors". The Oregonian. October 16, 2014
- ^ Schubert, E. Fred (2006) Light-emitting diodes (2nd ed.), Cambridge University Press. ISBN 0-521-86538-7 pp. 16–17
- ^ Maruska, H. (2005). "A Brief History of GaN Blue Light-Emitting Diodes". LIGHTimes Online – LED Industry News. Archived June 11, 2012, at the Wayback Machine
- ^ Major Business and Product Milestones. Cree.com. Retrieved on March 16, 2012. Archived April 13, 2011, at the Wayback Machine
- ^ Edmond, John A.; Kong, Hua-Shuang; Carter, Calvin H. (1993-04-01). "Blue LEDs, UV photodiodes and high-temperature rectifiers in 6H-SiC". Physica B: Condensed Matter. 185 (1): 453–460. Bibcode:1993PhyB..185..453E. doi:10.1016/0921-4526(93)90277-D. ISSN 0921-4526.
- ^ "History & Milestones". Cree.com. Cree. Archived from the original on February 16, 2017. Retrieved 2015-09-14.
- ^ "GaN-based blue light emitting device development by Akasaki and Amano" (PDF). Takeda Award 2002 Achievement Facts Sheet. The Takeda Foundation. April 5, 2002. Retrieved November 28, 2007.
- ^ Moustakas, Theodore D. U.S. patent 5686738A "Highly insulating monocrystalline gallium nitride thin films" Issue date: March 18, 1991
- ^ Brown, Joel (7 December 2015). "BU Wins $13 Million in Patent Infringement Suit". BU Today. Retrieved 7 December 2015.
- ^ Nakamura, S.; Mukai, T.; Senoh, M. (1994). "Candela-Class High-Brightness InGaN/AlGaN Double-Heterostructure Blue-Light-Emitting-Diodes". Applied Physics Letters. 64 (13): 1687. Bibcode:1994ApPhL..64.1687N. doi:10.1063/1.111832.
- ^ Nakamura, Shuji. "Development of the Blue Light-Emitting Diode". SPIE Newsroom. Retrieved 28 September 2015.
- ^ Iwasa, Naruhito; Mukai, Takashi and Nakamura, Shuji U.S. patent 5,578,839 "Light-emitting gallium nitride-based compound semiconductor device" Issue date: November 26, 1996
- ^ Fred Schubert, E. (January 2006). Light-Emitting Diodes (2nd Edition, 2006). E. Fred Schubert. ISBN 978-0-9863826-1-1.
- ^ "Professor Shuji Nakamura was key to the Invention of Blu-Ray Technology". University of California, Santa Barbara. 12 January 2023. Archived from the original on 24 March 2023. Retrieved 4 June 2023.
- ^ "Dr. Shuji Nakamura". National Academy of Engineering. Archived from the original on 11 April 2019. Retrieved 4 June 2023.
- ^ 2006 Millennium technology prize awarded to UCSB's Shuji Nakamura. Ia.ucsb.edu (June 15, 2006). Retrieved on August 3, 2019.
- ^ Overbye, Dennis (7 October 2014). "Nobel Prize in Physics". The New York Times.
- ^ Dadgar, A.; Alam, A.; Riemann, T.; Bläsing, J.; Diez, A.; Poschenrieder, M.; Strassburg, M.; Heuken, M.; Christen, J.; Krost, A. (2001). "Crack-Free InGaN/GaN Light Emitters on Si(111)". Physica Status Solidi A. 188: 155–158. doi:10.1002/1521-396X(200111)188:1<155::AID-PSSA155>3.0.CO;2-P.
- ^ Dadgar, A.; Poschenrieder, M.; BläSing, J.; Fehse, K.; Diez, A.; Krost, A. (2002). "Thick, crack-free blue light-emitting diodes on Si(111) using low-temperature AlN interlayers and in situ Si\sub x]N\sub y] masking". Applied Physics Letters. 80 (20): 3670. Bibcode:2002ApPhL..80.3670D. doi:10.1063/1.1479455.
- ^ "Success in research: First gallium-nitride LED chips on silicon in pilot stage" (PDF). Archived from the original (PDF) on September 15, 2012. Retrieved 2012-09-15.. www.osram.de, January 12, 2012.
- ^ Lester, Steve (2014) Role of Substrate Choice on LED Packaging Archived July 12, 2014, at the Wayback Machine. Toshiba America Electronic Components.
- ^ "GaN on Silicon". Cambridge Centre for Gallium Nitride. Gan.msm.cam.ac.uk. Retrieved July 31, 2018.
- ^ Bush, Steve (June 30, 2016). "Toshiba gets out of GaN-on-Si LEDs". Electronics Weekly. Retrieved July 31, 2018.
- ^ Nunoue, Shin-ya; Hikosaka, Toshiki; Yoshida, Hisashi; Tajima, Jumpei; Kimura, Shigeya; Sugiyama, Naoharu; Tachibana, Koichi; Shioda, Tomonari; Sato, Taisuke; Muramoto, Eiji; Onomura, Masaaki (2013). "LED manufacturing issues concerning gallium nitride-on-silicon (GaN-on-Si) technology and wafer scale up challenges". 2013 IEEE International Electron Devices Meeting. pp. 13.2.1–13.2.4. doi:10.1109/IEDM.2013.6724622. ISBN 978-1-4799-2306-9. S2CID 23448056.
- ^ Wright, Maury (May 2, 2016). "Samsung's Tarn reports progress in CSP and GaN-on-Si LEDs". LEDs Magazine.
- ^ "Increasing the Competitiveness of the GaN-on-silicon LED". Compound Semiconductor (30 March 2016).
- ^ "Samsung To Focus on Silicon-based LED Chip Technology in 2015". LED Inside (17 March 2015).
- ^ Keeping, Steven. (2013-01-15) "Material and Manufacturing Improvements". DigiKey. Retrieved on 2018-07-31.
- ^ Keeping, Steven (December 12, 2014) "Manufacturers Shift Attention to Light Quality to Further LED Market Share Gains". DigiKey. Retrieved July 31, 2018.
- ^ Keeping, Steven. (September 24, 2013). "Will Silicon Substrates Push LED Lighting Into the Mainstream?". DigiKey. Retrieved July 31, 2018.
- ^ Keeping, Steven (March 24, 2015). "Improved Silicon-Substrate LEDs Address High Solid-State Lighting Costs". DigiKey. Retrieved July 31, 2018.
- ^ "Development of the Nano-Imprint Equipment ST50S-LED for High-Brightness LED". Toshiba Machine (May 18, 2011). Retrieved July 31, 2018.
- ^ "The use of sapphire in mobile device and LED industries: Part 2" Archived July 29, 2018, at the Wayback Machine. Solid State Technology (September 26, 2017). Retrieved July 31, 2018.
- ^ "Epitaxy". Applied Materials. Retrieved July 31, 2018.
- ^ a b Lester, Steve, Role of Substrate Choice on LED Pacakaging (PDF), Toshiba America Electronic Components, archived from the original (PDF) on 12 July 2014
- ^ Semiengineering: MOCVD vendors eye new apps
- ^ Izotov, Sergey; Sitdikov, Anton; Soldatkin, Vasily; Tuev, Vasily; Olisovets, Artem (2014). "Study of Phosphors for White LEDs". Procedia Technology. 18: 14–18. doi:10.1016/j.protcy.2014.11.005.
- ^ "Haitz's law". Nature Photonics. 1 (1): 23. 2007. Bibcode:2007NaPho...1...23.. doi:10.1038/nphoton.2006.78.
- ^ "List of Top 10 LED light manufacturer in China". Archived from the original on 9 October 2014.
- ^ Morris, Nick (1 June 2006). "LED there be light, Nick Morris predicts a bright future for LEDs". Electrooptics.com. Archived from the original on November 23, 2011. Retrieved March 4, 2009.
- ^ "The LED Illumination Revolution". Forbes. February 27, 2008.
- ^ "The Nobel Prize in Physics 2014" (press release). Nobel Prize Committee, 7 October 2014
- ^ "Cree First to Break 300 Lumens-Per-Watt Barrier". Archived July 28, 2018, at the Wayback Machine. Cree.com (Match 26, 2014). Retrieved July 31, 2018.
- ^ LM301B | SAMSUNG LED | Samsung LED Global Website. Samsung.com. Retrieved on 2018-07-31.
- ^ Samsung Achieves 220 Lumens per Watt with New Mid-Power LED Package. Samsung.com (2017-06-16). Retrieved on 2018-07-31.
- ^ LED breakthrough promises ultra-efficient luminaires | Lux-n-Lum.Retrieved on 2018-04-06.
- ^ "White LEDs with super-high luminous efficacy could satisfy all general lighting needs". phys.org.
- ^ LED bulb efficiency expected to continue improving as cost declines. U.S. Energy Information Administration (March 19, 2014)
- ^ LED Lighting: Technology and Perception. John Wiley & Sons. February 9, 2015. ISBN 978-3-527-41212-9.
- ^ "Hermetic Polymer-Free White LEDs for Harsh Environments".
- ^ From LED to Solid State Lighting: Principles, Materials, Packaging, Characterization, and Applications. John Wiley & Sons. September 28, 2021. ISBN 978-1-118-88147-7.
- ^ Introduction to the Light-Emitting Diode: Real Applications for Industrial Engineers. Springer. May 12, 2023. ISBN 978-3-031-30716-4.
- ^ a b Reliability of Organic Compounds in Microelectronics and Optoelectronics: From Physics-of-Failure to Physics-of-Degradation. Springer. January 31, 2022. ISBN 978-3-030-81576-9.
- ^ From LED to Solid State Lighting: Principles, Materials, Packaging, Characterization, and Applications. John Wiley & Sons. September 28, 2021. ISBN 978-1-118-88147-7.
- ^ Fundamentals of Solid-State Lighting: LEDs, OLEDs, and Their Applications in Illumination and Displays. CRC Press. June 3, 2014. ISBN 978-1-4665-6112-0.
- ^ a b LED Packaging for Lighting Applications: Design, Manufacturing, and Testing. John Wiley & Sons. July 5, 2011. ISBN 978-0-470-82840-3.
- ^ a b LED Lighting: Technology and Perception. John Wiley & Sons. February 9, 2015. ISBN 978-3-527-41212-9.
- ^ Chung, Woon Jin; Nam, Yoon Hee (2020). "Review—A Review on Phosphor in Glass as a High Power LED Color Converter". ECS Journal of Solid State Science and Technology. 9 (1): 016010. Bibcode:2020JSSST...9a6010C. doi:10.1149/2.0142001JSS.
- ^ "Philips LED 60W 806lm Retrofit with Remote Phosphor". lamptech.co.uk. Retrieved 9 January 2022.
- ^ Light-Emitting Diodes (4th Edition, 2023). E. Fred Schubert. March 11, 2023. ISBN 978-0-9863826-7-3.
- ^ Reliability Investigation of LED Devices for Public Light Applications. Elsevier. March 9, 2017. ISBN 978-0-08-101092-1.
- ^ Solid State Lighting Reliability: Components to Systems. Springer. September 6, 2012. ISBN 978-1-4614-3067-4.
- ^ Solid State Lighting Reliability Part 2: Components to Systems. Springer. July 11, 2017. ISBN 978-3-319-58175-0.
- ^ Light-Emitting Diodes (2nd Edition, 2006). E. Fred Schubert. January 2006. ISBN 978-0-9863826-1-1.
- ^ Nitride Semiconductor Light-Emitting Diodes (LEDs): Materials, Technologies, and Applications. Woodhead. October 24, 2017. ISBN 978-0-08-101943-6.
- ^ LED Lighting: Technology and Perception. John Wiley & Sons. February 9, 2015. ISBN 978-3-527-41212-9.
- ^ Fundamentals of Solid-State Lighting: LEDs, OLEDs, and Their Applications in Illumination and Displays. CRC Press. June 3, 2014. ISBN 978-1-4665-6112-0.
- ^ III-Nitride Based Light Emitting Diodes and Applications. Springer. May 18, 2017. ISBN 978-981-10-3755-9.
- ^ "New Glass-Based Phosphors for White Light-Emitting Diodes".
- ^ From LED to Solid State Lighting: Principles, Materials, Packaging, Characterization, and Applications. John Wiley & Sons. September 28, 2021. ISBN 978-1-118-88147-7.
- ^ LED Lighting: Technology and Perception. John Wiley & Sons. February 9, 2015. ISBN 978-3-527-41212-9.
- ^ Coxworth, Ben (2024-06-18). "All-in-one polychromatic LEDs replace RGB for radically sharper screens". New Atlas. Retrieved 2024-06-21.