Urban heat island
![]() | It has been suggested that Urban dust dome be merged into this article. (Discuss) Proposed since October 2023. |


Urban areas usually experience the urban heat island (UHI) effect, that is, they are significantly warmer than surrounding rural areas. The temperature difference is usually larger at night than during the day,[1] and is most apparent when winds are weak, under block conditions, noticeably during the summer and winter. The main cause of the UHI effect is from the modification of land surfaces while waste heat generated by energy usage is a secondary contributor.[2][3][4] A study has shown that heat islands can be affected by proximity to different types of land cover, so that proximity to barren land causes urban land to become hotter and proximity to vegetation makes it cooler.[5] As a population center grows, it tends to expand its area and increase its average temperature. The term heat island is also used; the term can be used to refer to any area that is relatively hotter than the surrounding, but generally refers to human-disturbed areas.[6] Urban areas occupy about 0.5% of the Earth's land surface but host more than half of the world's population.[7]
Monthly rainfall is greater downwind of cities, partially due to the UHI. Increases in heat within urban centers increases the length of growing seasons and decreases the occurrence of weak tornadoes. The UHI decreases air quality by increasing the production of pollutants such as ozone, and decreases water quality as warmer waters flow into area streams and put stress on their ecosystems.
Not all cities have a distinct urban heat island, and the heat island characteristics depend strongly on the background climate of the area in which the city is located.[8] The impact in a city can change a lot based on its local environment. Heat can be reduced by tree cover and green space which act as sources of shade and promote evaporative cooling.[9] Other options include green roofs, passive daytime radiative cooling applications, and the use of lighter-colored surfaces and less absorptive building materials. These reflect more sunlight and absorb less heat.[10][11][12]
Climate change is not the cause of urban heat islands but it is causing more frequent and more intense heat waves which in turn amplify the urban heat island effect in cities.[13]: 993 Compact, dense urban development may increase the urban heat island effect, leading to higher temperatures and increased exposure.[14]
Definition
[edit]A definition of urban heat island is: "The relative warmth of a city compared with surrounding rural areas."[15]: 2926 This relative warmth is caused by "heat trapping due to land use, the configuration and design of the built environment, including street layout and building size, the heat-absorbing properties of urban building materials, reduced ventilation, reduced greenery and water features, and domestic and industrial heat emissions generated directly from human activities".[15]: 2926
Description
[edit]

Diurnal variability
[edit]
Throughout the daytime, particularly when the skies are cloudless, urban surfaces are warmed by the absorption of solar radiation. Surfaces in the urban areas tend to warm faster than those of the surrounding rural areas. By virtue of their high heat capacities, urban surfaces act as a reservoir of heat energy. For example, concrete can hold roughly 2,000 times as much heat as an equivalent volume of air.[citation needed] As a result, high daytime surface temperatures within the UHI can be easily seen via thermal remote sensing. As is often the case with daytime heating, this warming also has the effect of generating convective winds within the urban boundary layer. At night, the situation reverses. The absence of solar heating leads to the decrease of atmospheric convection and the stabilization of urban boundary layer. If enough stabilization occurs, an inversion layer is formed. This traps urban air near the surface, keeping surface air warm from the still-warm urban surfaces, resulting in warmer nighttime air temperatures within the UHI.
Generally speaking, the difference in temperature between the urban and surrounding rural area is more pronounced at night than in daytime.[16] For example, in the United States, the temperature in urban areas tends to be warmer than the surrounding area by about 1-7° F during the daytime, and about 2-5° F warmer at night.[17] However, the difference is more pronounced during the day in arid climates such as those in southeastern China and Taiwan.[18][19] Studies have shown that diurnal variability is impacted by several factors including local climate and weather, seasonality, humidity, vegetation, surfaces, and materials in the built environment.[20][17][21][22]
Seasonal variability
[edit]Seasonal variability is less well understood than diurnal variability of the urban heat island temperature difference.[23] Complex relationships between precipitation, vegetation, solar radiation, and surface materials in various local climate zones play interlocking roles that influence seasonal patterns of temperature variation in a particular urban heat island.[23][24][25][26]
Measurements and predictions
[edit]Urban Heat Island Index (UHII)
[edit]One method to quantify the UHI effect within urban areas is the UHI Index created by the Californian EPA in 2015. It compares the temperature of a surveyed area and rural reference points upwind from the surveyed area, at a height of two meters above ground level. The difference in temperature in degree Celsius is taken hourly and differences with an increased urban temperature compared to the reference points are summed up, creating an amount of degree Celsius hours, which is the UHI Index of the surveyed area. The measure of Celsius hours might be averaged over many days, but is specified as Celsius hours per averaged day.[27][28][29]
The index was created to estimate the expected use of air conditioning and resulting greenhouse gas emissions in California.[28] The index does not consider values of or differences in wind-speed, humidity, or solar influx, which might influence perceived temperature or the operation of air conditioners.[29]
Models and simulations
[edit]If a city or town has a good system of taking weather observations the UHI can be measured directly.[30] An alternative is to use a complex simulation of the location to calculate the UHI, or to use an approximate empirical method.[31][32] Such models allow the UHI to be included in estimates of future temperatures rises within cities due to climate change.
Leonard O. Myrup published the first comprehensive numerical treatment to predict the effects of the urban heat island (UHI) in 1969.[33] The heat island effect was found to be the net result of several competing physical processes. In general, reduced evaporation in the city center and the thermal properties of the city building and paving materials are the dominant parameters.[33] Modern simulation environments include ENVI-met, which simulates all interactions between building and ground surfaces, plants and ambient air.[34]
Causes
[edit]

There are several causes of an urban heat island (UHI); for example, dark surfaces absorb significantly more solar radiation, which causes urban concentrations of roads and buildings to heat more than suburban and rural areas during the day;[2] materials commonly used in urban areas for pavement and roofs, such as concrete and asphalt, have significantly different thermal bulk properties (including heat capacity and thermal conductivity) and surface radiative properties (albedo and emissivity) than the surrounding rural areas. This causes a change in the energy budget of the urban area, often leading to higher temperatures than surrounding rural areas.[35]
Pavements, parking lots, roads or, more generally speaking transport infrastructure, contribute significantly to the urban heat island effect.[36] For example, pavement infrastructure is a main contributor to urban heat during summer afternoons in Phoenix, United States.[36]
Another major reason is the lack of evapotranspiration (for example, through lack of vegetation) in urban areas.[37] The U.S. Forest Service found in 2018 that cities in the United States are losing 36 million trees each year.[38] With a decreased amount of vegetation, cities also lose the shade and evaporative cooling effect of trees.[39][40]
Other causes of a UHI are due to geometric effects. The tall buildings within many urban areas provide multiple surfaces for the reflection and absorption of sunlight, increasing the efficiency with which urban areas are heated. This is called the "urban canyon effect". Another effect of buildings is the blocking of wind, which also inhibits cooling by convection and prevents pollutants from dissipating. Waste heat from automobiles, air conditioning, industry, and other sources also contributes to the UHI.[4][41][42]
High levels of pollution in urban areas can also increase the UHI, as many forms of pollution change the radiative properties of the atmosphere.[35] UHI not only raises urban temperatures but also increases ozone concentrations because ozone is a greenhouse gas whose formation will accelerate with the increase of temperature.[43]
Climate change as an amplifier
[edit]Climate change is not a cause but an amplifier of the urban heat island effect. The IPCC Sixth Assessment Report from 2022 summarized the available research accordingly: "Climate change increases heat stress risks in cities [...] and amplifies the urban heat island across Asian cities at 1.5°C and 2°C warming levels, both substantially larger than under present climates [...]."[44]: 66
The report goes on to say: "In a warming world, increasing air temperature makes the urban heat island effect in cities worse. One key risk is heatwaves in cities that are likely to affect half of the future global urban population, with negative impacts on human health and economic productivity."[13]: 993
There are unhelpful interactions between heat and built infrastructure: These interactions increase the risk of heat stress for people living in cities.[13]: 993
Impacts
[edit]
On weather and climate
[edit]Aside from the effect on temperature, UHIs can produce secondary effects on local meteorology, including the altering of local wind patterns, the development of clouds and fog, the humidity, and the rates of precipitation.[45] The extra heat provided by the UHI leads to greater upward motion, which can induce additional shower and thunderstorm activity. In addition, the UHI creates during the day a local low pressure area where relatively moist air from its rural surroundings converges, possibly leading to more favorable conditions for cloud formation.[46] Rainfall rates downwind of cities are increased between 48% and 116%. Partly as a result of this warming, monthly rainfall is about 28% greater between 20 to 40 miles (32 to 64 km) downwind of cities, compared with upwind.[47] Some cities show a total precipitation increase of 51%.[48]
One study concluded that cities change the climate in area two–four times larger than their own area.[49] One 1999 comparison between urban and rural areas proposed that urban heat island effects have little influence on global mean temperature trends.[50] Others suggested that urban heat islands affect global climate by impacting the jet stream.[51]
On human health
[edit]
UHIs have the potential to directly influence the health and welfare of urban residents. As UHIs are characterized by increased temperature, they can potentially increase the magnitude and duration of heat waves within cities. The number of individuals exposed to extreme temperatures is increased by the UHI-induced warming.[52] The nighttime effect of UHIs can be particularly harmful during a heat wave, as it deprives urban residents of the cool relief found in rural areas during the night.[53]
Increased temperatures have been reported to cause heat illnesses, such as heat stroke, heat exhaustion, heat syncope, and heat cramps.[54]
Extreme heat is the deadliest form of weather in the U.S. Per a study by Professor Terri Adams-Fuller, heat waves kill more people in the U.S. than hurricanes, floods, and tornadoes combined.[55] These heat illnesses are more common within medium-to-large metro areas than the rest of the U.S., largely in part due to UHIs. Heat illnesses can also be compounded when combined with air pollution which is common in many urban areas.
Heat exposure can have adverse effects on mental health. Increases in temperature can contribute to increased aggression, as well as more cases of domestic violence and substance abuse.[56] Greater heat can also negatively impact school performance and education. According to a study by Hyunkuk Cho of Yeungnam University, an increased number of days with extreme heat each year correlates to a decrease in student test scores.[57]
High UHI intensity correlates with increased concentrations of air pollutants that gathered at night, which can affect the next day's air quality.[58] These pollutants include volatile organic compounds, carbon monoxide, nitrogen oxides, and particulate matter.[59] The production of these pollutants combined with the higher temperatures in UHIs can quicken the production of ozone.[58] Ozone at surface level is considered to be a harmful pollutant.[58] Studies suggest that increased temperatures in UHIs can increase polluted days but also note that other factors (e.g. air pressure, cloud cover, wind speed) can also have an effect on pollution.[58]
Studies from Hong Kong have found that areas of the city with poorer outdoor urban air ventilation tended to have stronger urban heat island effects[60] and had significantly higher all-cause mortality[61] compared to areas with better ventilation. Another study employing advanced statistical methods in Babol city, Iran, revealed a significant increase in Surface Urban Heat Island Intensity (SUHII) from 1985 to 2017, influenced by both geographic direction and time. This research, enhancing the understanding of SUHII's spatial and temporal variations, emphasizes the need for precise urban planning to mitigate the health impacts of urban heat islands.[62] Surface UHI's are more prominent during the day and are measured using the land surface temperature and remote sensing.[63]
On water bodies and aquatic organisms
[edit]UHIs also impair water quality. Hot pavement and rooftop surfaces transfer their excess heat to stormwater, which then drains into storm sewers and raises water temperatures as it is released into streams, rivers, ponds, and lakes. Additionally, increased urban water body temperatures lead to a decrease in biodiversity in the water.[64] For example, in August 2001, rains over Cedar Rapids, Iowa led to a 10.5 °C (18.9 °F) rise in the nearby stream within one hour, resulting in a fish kill which affected an estimated 188 fish.[65] Since the temperature of the rain was comparatively cool, the deaths could be attributed to the hot pavement of the city. Similar events have been documented across the American Midwest, as well as Oregon and California.[66] Rapid temperature changes can be stressful to aquatic ecosystems.[67]
With the temperature of the nearby buildings sometimes reaching a difference of over 50 °F (28 °C) from the near-surface air temperature, precipitation warms rapidly, and run-off into nearby streams, lakes and rivers (or other bodies of water) to provide excessive thermal pollution. The increase in thermal pollution has the potential to increase water temperature by 20 to 30 °F (11 to 17 °C). This increase causes the fish species inhabiting the body of water to undergo thermal stress and shock due to the rapid change in temperature of their habitat.[68]
Permeable pavements may reduce these effects by percolating water through the pavement into subsurface storage areas where it can be dissipated through absorption and evaporation.[69]
On animals
[edit]Species that are good at colonizing can use conditions provided by urban heat islands to thrive in regions outside of their normal range. Examples of this include the grey-headed flying fox (Pteropus poliocephalus) and the common house gecko (Hemidactylus frenatus).[70] Grey-headed flying foxes, found in Melbourne, Australia, colonized urban habitats following the increase in temperatures there. Increased temperatures, causing warmer winter conditions, made the city more similar in climate to the more northerly wildland habitat of the species.
With temperate climates, urban heat islands will extend the growing season, therefore altering breeding strategies of inhabiting species.[70] This can be best observed in the effects that urban heat islands have on water temperature (see effects on water bodies).
Urban heat islands caused by cities have altered the natural selection process.[70] Selective pressures like temporal variation in food, predation and water are relaxed causing a new set of selective forces to roll out. For example, within urban habitats, insects are more abundant than in rural areas. Insects are ectotherms. This means that they depend on the temperature of the environment to control their body temperature, making the warmer climates of the city perfect for their ability to thrive. A study done in Raleigh, North Carolina conducted on Parthenolecanium quercifex (oak scales), showed that this particular species preferred warmer climates and were therefore found in higher abundance in urban habitats than on oak trees in rural habitats. Over time spent living in urban habitats, they have adapted to thrive in warmer climates than in cooler ones.[71]
On energy usage for cooling
[edit]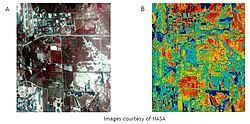
Another consequence of urban heat islands is the increased energy required for air conditioning and refrigeration in cities that are in comparatively hot climates. The heat island effect costs Los Angeles about US$ 100 million per year in energy (in the year 2000).[72] Through the implementation of heat island reduction strategies, significant annual net energy savings have been calculated for northern locations such as Chicago, Salt Lake City, and Toronto.[73]
Every year in the U.S. 15% of energy goes towards the air conditioning of buildings in these urban heat islands. It was reported in 1998 that "the air conditioning demand has risen 10% within the last 40 years."[74]
Increases in air conditioning use also serve to worsen the effects of UHIs at night. While cooler nights are often a reprieve from heat waves during the day, the residual heat created by the use of air conditioning systems can lead to higher nighttime temperatures. According to a study by Professor Francisco Salamanca Palou and colleagues, this residual heat can cause nighttime increases of up to 1°Celsius in urban areas.[75] Increased energy use from air conditioners also contributes to carbon emissions, which doubly exacerbates the effects of UHIs.
Options for reducing heat island effects
[edit]
Strategies to improve urban resilience by reducing excessive heat in cities include: Planting trees in cities, cool roofs (painted white or with reflective coating) and light-coloured concrete, green infrastructure (including green roofs), passive daytime radiative cooling.[76]
The temperature difference between urban areas and the surrounding suburban or rural areas can be as much as 5 °C (9.0 °F). Nearly 40 percent of that increase is due to the prevalence of dark roofs, with the remainder coming from dark-colored pavement and the declining presence of vegetation. The heat island effect can be counteracted slightly by using white or reflective materials to build houses, roofs, pavements, and roads, thus increasing the overall albedo of the city.[77]
Concentric expansion of cities is unfavorable in terms of the urban heat island phenomenon. It is recommended to plan the development of cities in strips, consistent with the hydrographic network, taking into account green areas with various plant species.[78] In this way, it was planned to build urban settlements stretching over large areas, e.g. Kielce, Szczecin and Gdynia in Poland, Copenhagen in Denmark and Hamburg, Berlin and Kiel in Germany.
Planting trees in cities
[edit]Planting trees around the city can be another way of increasing albedo and decreasing the urban heat island effect. It is recommended to plant deciduous trees because they can provide many benefits such as more shade in the summer and not blocking warmth in winter.[79] Trees are a necessary feature in combating most of the urban heat island effect because they reduce air temperatures by 10 °F (5.6 °C),[80] and surface temperatures by up to 20–45 °F (11–25 °C).[81] Another benefit of having trees in a city is that trees also help fight global warming by absorbing CO2 from the atmosphere.
Cool roofs and light-coloured concrete
[edit]Painting rooftops white has become a common strategy to reduce the heat island effect.[82] In cities, there are many dark colored surfaces that absorb the heat of the sun in turn lowering the albedo of the city.[82] White rooftops allow high solar reflectance and high solar emittance, increasing the albedo of the city or area the effect is occurring.[82]
Additionally, covering rooftops with a reflective coating, has shown to be an effective measure to reduce solar heat gain. A study led by Oscar Brousse from University College London, which simulated the impact of various cooling measures in London found that rooftops, which were either painted white or had reflective coating, proved to be the most effective solution for reducing outdoor temperatures at the pedestrian level, outperforming solar panels, green roofs, and tree cover. The study simulated the impact of various cooling measures in London during a 2018 heatwave, finding that the so-called cool roofs could reduce average outdoor temperatures by 1.2 °C, and up to 2 °C in certain areas. In comparison, additional tree cover reduced temperatures by 0.3 °C and solar panels by 0.5° C.[83]
Relative to remedying the other sources of the problem, replacing dark roofing requires the least amount of investment for the most immediate return. A cool roof made from a reflective material such as vinyl reflects at least 75 percent of the sun's rays, and emit at least 70 percent of the solar radiation absorbed by the building envelope. Asphalt built-up roofs (BUR), by comparison, reflect 6 percent to 26 percent of solar radiation.[84]
Using light-colored concrete has proven effective in reflecting up to 50% more light than asphalt and reducing ambient temperature.[85] A low albedo value, characteristic of black asphalt, absorbs a large percentage of solar heat creating warmer near-surface temperatures. Paving with light-colored concrete, in addition to replacing asphalt with light-colored concrete, communities may be able to lower average temperatures.[86] However, research into the interaction between reflective pavements and buildings has found that, unless the nearby buildings are fitted with reflective glass, solar radiation reflected off light-colored pavements can increase building temperatures, increasing air conditioning demands.[87][88]
There are specific paint formulations for daytime radiative cooling that reflect up to 98.1% of sunlight.[89][90]
Green infrastructure
[edit]
Green roofs are excellent insulators during the warm weather months and the plants cool the surrounding environment. Plants can improve air quality as they absorb carbon dioxide and concomitantly produce oxygen.[91] Green roofs can also have positive impacts on stormwater management and energy consumption.[92] Cost can be a barrier to implementing a green roof.[93][94] Several factors influence the cost of a green roof, including design and soil depth, location, and the price of labor and equipment in that market, which is typically lower in more developed markets where there is more experience designing and installing green roofs.[95] The individualized context of each green roof presents a challenge for making broad comparisons and assessments, and focusing only on monetary costs may leave out the social, environmental, and public health benefits green roofs provide.[94] Global comparisons of green roof performance are further challenged by the lack of a shared framework for making such comparisons.[94]
Stormwater management is another option to help mitigate the effect of the urban heat island. Stormwater management is the controlling the water produced by the storm in a way that protects property and infrastructure.[96] Urban infrastructure like streets, sidewalks, and parking lots do not allow for water to penetrate into the earth's surface causing water to flood. By using stormwater management, you can control the flow of the water in ways that can mitigate UHI effect. One way is using a stormwater management technique called pervious pavement system (PPS). This technique has been used in over 30 countries and found to be successful in stormwater management and UHI mitigation. The PPS allows water to flow through the pavement allowing for the water to be absorbed causing the area to be cooled by evaporation.[97]
Green parking lots use vegetation and surfaces other than asphalt to limit the urban heat island effect.

Passive daytime radiative cooling
[edit]A passive daytime radiative cooling roof application can double the energy savings of a white roof, attributed to high solar reflectance and thermal emittance in the infrared window,[103] with the highest cooling potential in hot and dry cities such as Phoenix and Las Vegas.[104] When installed on roofs in dense urban areas, passive daytime radiative cooling panels can significantly lower outdoor surface temperatures at the pedestrian level.[11][12]
Society and culture
[edit]History of research
[edit]The phenomenon was first investigated and described by Luke Howard in the 1810s, although he was not the one to name the phenomenon.[105] A description of the very first report of the UHI by Luke Howard said that the urban center of London was warmer at night than the surrounding countryside by 2.1 °C (3.7 °F).[106]
Investigations of the urban atmosphere continued throughout the nineteenth century. Between the 1920s and the 1940s, researchers in the emerging field of local climatology or microscale meteorology in Europe, Mexico, India, Japan, and the United States pursued new methods to understand the phenomenon.
In 1929, Albert Peppler used the term in a German publication believed to be the first instance of an equivalent to urban heat island: städtische Wärmeinsel (which is urban heat island in German).[107] Between 1990 and 2000, about 30 studies were published annually; by 2010, that number had increased to 100, and by 2015, it was more than 300.[108]
Leonard O. Myrup published the first comprehensive numerical treatment to predict the effects of the urban heat island (UHI) in 1969.[33] His paper surveys UHI and criticizes then-existing theories as being excessively qualitative.
Aspects of social inequality
[edit]Some studies suggest that the effects of UHIs on health may be disproportionate, since the impacts may be unevenly distributed based on a variety of factors such as age,[59][109] ethnicity and socioeconomic status.[110] This raises the possibility of health impacts from UHIs being an environmental justice issue. Studies have shown that communities of color in the United States have been disproportionately affected by UHI.[111][112][113]
There is a correlation between neighborhood income and tree canopy cover.[114] Low-income neighborhoods tend to have significantly fewer trees than neighborhoods with higher incomes.[115] Researchers hypothesized that less-well-off neighborhoods do not have the financial resources to plant and maintain trees. Affluent neighborhoods can afford more trees, on "both public and private property."[116] One reason for this discrepancy is that wealthier homeowners and communities can afford more land, which can be kept open as green space, whereas poorer housing often takes the form of rentals, where landowners try to maximize their profit by putting as much housing density as possible on their land.[117]
Chief heat officers
[edit]Beginning in the 2020s, a number of cities worldwide began creating Chief Heat Officer positions to organize and manage work counteracting the urban heat island effect.[118][119]
Examples
[edit]![]() | The examples and perspective in this article deal primarily with the United States and do not represent a worldwide view of the subject. (March 2023) |
United States
[edit]Bill S.4280,[120] introduced to the U.S. Senate in 2020, would authorize the National Integrated Heat Health Information System Interagency Committee (NIHHIS) to tackle extreme heat in the United States.[121] Successful passage of this legislation would fund NIHHIS for five years and would instate a $100 million grant program within NIHHIS to encourage and fund urban heat reduction projects, including those using cools roofs and pavements and those improving HVAC systems. As of July 22, 2020 the bill has not moved past introduction to Congress.
The city of New York determined that the cooling potential per area was highest for street trees, followed by living roofs, light covered surface, and open space planting. From the standpoint of cost effectiveness, light surfaces, light roofs, and curbside planting have lower costs per temperature reduction.[122]
Los Angeles
[edit]A hypothetical "cool communities" program in Los Angeles has projected in 1997 that urban temperatures could be reduced by approximately 3 °C (5 °F) after planting ten million trees, reroofing five million homes, and painting one-quarter of the roads at an estimated cost of US$1 billion, giving estimated annual benefits of US$170 million from reduced air-conditioning costs and US$360 million in smog related health savings.[79]
In a case study of the Los Angeles Basin in 1998, simulations showed that even when trees are not strategically placed in these urban heat islands, they can still aid in minimization of pollutants and energy reduction. It is estimated that with this wide-scale implementation, the city of Los Angeles can annually save $100M with most of the savings coming from cool roofs, lighter colored pavement, and the planting of trees. With a citywide implementation, added benefits from the lowering smog-level would result in at least one billion dollars of saving per year.[74]
Los Angeles TreePeople is an example of how tree planting can empower a community. Tree people provides the opportunity for people to come together, build capacity, community pride and the opportunity to collaborate and network with each other.[123]
Los Angeles has also begun to implement a Heat Action Plan to address the city's needs at a more granular level than the solutions provided by the state of California. The city uses the LA Equity Index in an effort to ensure that the effects of extreme heat are mitigated in an equitable manner.[124]
Virginia
[edit]In 2021, Climate Adaptation Planning Analysis (CAPA) received funding from the National Oceanic and Atmospheric Administration to conduct Heat Mapping across the United States.[125] Ten areas from Virginia - Abington, Arlington, Charlottesville, Farmville, Harrisonburg, Lynchburg, Petersburg, Richmond, Salem, Virginia Beach and Winchester - participated in the heat watch campaign. This campaign consisted of 213 Volunteers brought together by campaign organizers who made 490,423 Heat Measurements across 70 Routes total. After taking measurements throughout the day, equipment and data was sent back to CAPA where it was analyzed using machine learning algorithms. After analysis of the data, CAPA came back together with campaign organizers from each area to discuss potential plans for each town in the future.
New York
[edit]New York City implemented its "Cool Neighborhoods NYC" program in 2017 intending to mitigate the effects of extreme urban heat. One of the plan's ambitions was to increase funding for the city's Low-Income Home Energy Assistance Program. Specifically, the plan sought to increase funding for cooling solutions for lower-income families.[126]
See also
[edit]References
[edit]- ^ Phelan, Patrick E.; Kaloush, Kamil; Miner, Mark; Golden, Jay; Phelan, Bernadette; Silva, Humberto; Taylor, Robert A. (4 November 2015). "Urban Heat Island: Mechanisms, Implications, and Possible Remedies". Annual Review of Environment and Resources. 40 (1): 285–307. doi:10.1146/annurev-environ-102014-021155. S2CID 154497357.
- ^ a b Solecki, William D.; Rosenzweig, Cynthia; Parshall, Lily; Pope, Greg; Clark, Maria; Cox, Jennifer; Wiencke, Mary (2005). "Mitigation of the heat island effect in urban New Jersey". Global Environmental Change Part B: Environmental Hazards. 6 (1): 39–49. doi:10.1016/j.hazards.2004.12.002. S2CID 153841143.
- ^ United States Environmental Protection Agency (2008). Reducing urban heat islands: Compendium of strategies (Report). pp. 7–12.
- ^ a b Li, Y.; Zhao, X. (2012). "An empirical study of the impact of human activity on long-term temperature change in China: A perspective from energy consumption". Journal of Geophysical Research. 117 (D17): D17117. Bibcode:2012JGRD..11717117L. doi:10.1029/2012JD018132.
- ^ Mansourmoghaddam, Mohammad; Alavipanah, Seyed Kazem (2022). "Study and prediction of land surface temperature changes of Yazd city: assessing the proximity and changes of land cover". RS and GIS for Natural Resources. 12 (4): 1–27.
- ^ Glossary of Meteorology (2019). "Urban Heat Island". American Meteorological Society. Retrieved 2019-04-12.
- ^ Wang, K (February 6, 2017). "Comparing the diurnal and seasonal variabilities of atmospheric, and surface urban heat islands based on the Beijing Urban Meteorological Network". Journal of Geophysical Research: Atmospheres. 122 (4): 2131–2154. Bibcode:2017JGRD..122.2131W. doi:10.1002/2016JD025304.
- ^ T. Chakraborty and X. Lee (2019). "A simplified urban-extent algorithm to characterize surface urban heat islands on a global scale and examine vegetation control on their spatiotemporal variability". International Journal of Applied Earth Observation and Geoinformation. 74: 269–280. Bibcode:2019IJAEO..74..269C. doi:10.1016/j.jag.2018.09.015. S2CID 53715577.
- ^ Waldrop, M. Mitchell (19 October 2022). "What can cities do to survive extreme heat?". Knowable Magazine. doi:10.1146/knowable-101922-2. Retrieved 6 December 2022.
- ^ "Nature of Cities". Regeneration.org. Retrieved 2021-10-16.
- ^ a b Younes, Jaafar; Ghali, Kamel; Ghaddar, Nesreen (August 2022). "Diurnal Selective Radiative Cooling Impact in Mitigating Urban Heat Island Effect". Sustainable Cities and Society. 83: 103932. Bibcode:2022SusCS..8303932Y. doi:10.1016/j.scs.2022.103932. S2CID 248588547.
- ^ a b Khan, Ansar; Carlosena, Laura; Feng, Jie; Khorat, Samiran; Khatun, Rupali; Doan, Quang-Van; Santamouris, Mattheos (19 January 2022). "Optically Modulated Passive Broadband Daytime Radiative Cooling Materials Can Cool Cities in Summer and Heat Cities in Winter". Sustainability. 14 (3): 1110. doi:10.3390/su14031110. hdl:2454/46738.
- ^ a b c "Cities, Settlements and Key Infrastructure". Climate Change 2022 – Impacts, Adaptation and Vulnerability. 2023. pp. 907–1040. doi:10.1017/9781009325844.008. ISBN 978-1-009-32584-4.
- ^ Sharifi, Ayyoob (2020). "Trade-offs and conflicts between urban climate change mitigation and adaptation measures: A literature review". Journal of Cleaner Production. 276: 122813. Bibcode:2020JCPro.27622813S. doi:10.1016/j.jclepro.2020.122813. S2CID 225638176.
- ^ a b "Glossary". Climate Change 2022 – Impacts, Adaptation and Vulnerability. 2023. pp. 2897–2930. doi:10.1017/9781009325844.029. ISBN 978-1-009-32584-4.
- ^ "Urban Heat Islands | Center for Science Education". scied.ucar.edu. Retrieved 2024-07-02.
- ^ a b US EPA, OAR (2014-06-17). "Learn About Heat Islands". www.epa.gov. Retrieved 2024-07-01.
- ^ Zhou, Decheng; Zhang, Liangxia; Hao, Lu; Sun, Ge; Liu, Yongqiang; Zhu, Chao (2016). "Spatiotemporal trends of urban heat island effect along the urban development intensity gradient in China". Science of the Total Environment. 544: 617–626. Bibcode:2016ScTEn.544..617Z. doi:10.1016/j.scitotenv.2015.11.168. ISSN 0048-9697. PMID 26674691.
- ^ Liou, Yuei-An; Tran, Duy-Phien; Nguyen, Kim-Anh (2022-02-22). "Diurnal and Seasonal Characteristics of Surface Urban Heat Island in Taiwan". (CETA 2021) 2021 International Conference on Computer Engineering, Technologies and Applications. ISSN 2516-2314.
- ^ Amorim, Margarete Cristiane de Costa Trindade; Dubreuil, Vincent; Amorim, Amanda Trindade (2021). "Day and night surface and atmospheric heat islands in a continental and temperate tropical environment". Urban Climate. 38: 100918. Bibcode:2021UrbCl..3800918A. doi:10.1016/j.uclim.2021.100918. ISSN 2212-0955.
- ^ Shastri, Hiteshri; Barik, Beas; Ghosh, Subimal; Venkataraman, Chandra; Sadavarte, Pankaj (2017-01-09). "Flip flop of Day-night and Summer-Winter Surface Urban Heat Island Intensity in India". Scientific Reports. 7 (1): 40178. Bibcode:2017NatSR...740178S. doi:10.1038/srep40178. ISSN 2045-2322. PMC 5220321. PMID 28067276.
- ^ Mohajerani, Abbas; Bakaric, Jason; Jeffrey-Bailey, Tristan (2017-07-15). "The urban heat island effect, its causes, and mitigation, with reference to the thermal properties of asphalt concrete". Journal of Environmental Management. 197: 522–538. Bibcode:2017JEnvM.197..522M. doi:10.1016/j.jenvman.2017.03.095. ISSN 0301-4797. PMID 28412623.
- ^ a b Manoli, Gabriele; Fatichi, Simone; Bou-Zeid, Elie; Katul, Gabriel G. (2020-03-31). "Seasonal hysteresis of surface urban heat islands". Proceedings of the National Academy of Sciences. 117 (13): 7082–7089. Bibcode:2020PNAS..117.7082M. doi:10.1073/pnas.1917554117. ISSN 0027-8424. PMC 7132285. PMID 32184330.
- ^ Imhoff, Marc L.; Zhang, Ping; Wolfe, Robert E.; Bounoua, Lahouari (2010-03-15). "Remote sensing of the urban heat island effect across biomes in the continental USA". Remote Sensing of Environment. 114 (3): 504–513. Bibcode:2010RSEnv.114..504I. doi:10.1016/j.rse.2009.10.008. hdl:2060/20110015410. ISSN 0034-4257.
- ^ Cui, Yu Yan; Foy, Benjamin de (2012-05-01). "Seasonal Variations of the Urban Heat Island at the Surface and the Near-Surface and Reductions due to Urban Vegetation in Mexico City". Journal of Applied Meteorology and Climatology. 51 (5): 855–868. Bibcode:2012JApMC..51..855C. doi:10.1175/JAMC-D-11-0104.1. ISSN 1558-8424.
- ^ Han, Liying; Lu, Linlin; Fu, Peng; Ren, Chao; Cai, Meng; Li, Qingting (2023). "Exploring the seasonality of surface urban heat islands using enhanced land surface temperature in a semi-arid city". Urban Climate. 49: 101455. Bibcode:2023UrbCl..4901455H. doi:10.1016/j.uclim.2023.101455.
- ^ "Executive Summary: Creating and Mapping an Urban Heat Island Index for California" (PDF). CalEPA | California Environmental Protection Agency. October 2016. Archived (PDF) from the original on 2019-07-31. Retrieved 2024-07-24.
- ^ a b "Understanding the Urban Heat Island Index | CalEPA". CalEPA | California Environmental Protection Agency. Archived from the original on 2017-12-19. Retrieved 2024-07-24.
- ^ a b "Creating and Mapping an Urban Heat Island Index for California" (PDF). CalEPA | California Environmental Protection Agency. October 2016. Archived (PDF) from the original on 2019-07-31. Retrieved 2024-07-24.
- ^ Steeneveld, G.J. (2011). "Quantifying urban heat island effects and human comfort for cities of variable size and urban morphology in the Netherlands". Journal of Geophysical Research. 116 (D20): D20129. Bibcode:2011JGRD..11620129S. doi:10.1029/2011JD015988.
- ^ Kershaw, T. J.; Sanderson, M.; Coley, D.; Eames, M. (2010). "Estimation of the urban heat island for UK climate change projections". Building Services Engineering Research and Technology. 31 (3): 251–263. doi:10.1177/0143624410365033. hdl:10871/13934.
- ^ Theeuwes, N. E.; Steeneveld, G.J.; Ronda, R.J.; Holtslag, A.A.M. (2017). "A diagnostic equation for the daily maximum urban heat island effect for cities in northwestern Europe". International Journal of Climatology. 37 (1): 443–454. Bibcode:2017IJCli..37..443T. doi:10.1002/joc.4717. S2CID 131437962.
- ^ a b c Myrup, Leonard O. (1969). "A Numerical Model of the Urban Heat Island". Journal of Applied Meteorology. 8 (6): 908–918. Bibcode:1969JApMe...8..908M. doi:10.1175/1520-0450(1969)008<0908:ANMOTU>2.0.CO;2.
- ^ n.n. "ENVI-met-Alternativen für Mac — Altapps.net". de.altapps.net (in German). Retrieved 2022-06-01.
- ^ a b T. R. Oke (1982). "The energetic basis of the urban heat island". Quarterly Journal of the Royal Meteorological Society. 108 (455): 1–24. Bibcode:1982QJRMS.108....1O. doi:10.1002/qj.49710845502. S2CID 120122894.
- ^ a b Hoehne, Christopher G.; Chester, Mikhail V.; Sailor, David J.; King, David A. (4 July 2022). "Urban Heat Implications from Parking, Roads, and Cars: a Case Study of Metro Phoenix". Sustainable and Resilient Infrastructure. 7 (4): 272–290. Bibcode:2022SusRI...7..272H. doi:10.1080/23789689.2020.1773013. S2CID 225553384.
- ^ Kumar, Rahul; Mishra, Vimal; Buzan, Jonathan; Kumar, Rohini; Shindell, Drew; Huber, Matthew (25 October 2017). "Dominant control of agriculture and irrigation on urban heat island in India". Scientific Reports. 7 (1): 14054. Bibcode:2017NatSR...714054K. doi:10.1038/s41598-017-14213-2. PMC 5656645. PMID 29070866.
- ^ Larsson, Naomi (10 May 2018). "US cities losing 36 million trees a year, researchers find". The Guardian. Retrieved 10 May 2018.
- ^ Santos, Fabiane (23 August 2013). "Trees – the Natural Air Conditioners". Scientific Scribbles. University of Melbourne. Archived from the original on 2022-04-07.
- ^ Gorsevski, V.; Luvall, J.; Quattrochi, D.; Taha, H. (1998). "Air Pollution Prevention Through Urban Heat Island Mitigation: An Update on the Urban Heat Island Pilot Project" (PDF). Lawrence Berkeley National Lab. (LBNL). CiteSeerX 10.1.1.111.4921. LBNL-42736.
- ^ Sailor, D. J. (2011). "A review of methods for estimating anthropogenic heat and moisture emissions in the urban environment". International Journal of Climatology. 31 (2): 189–199. Bibcode:2011IJCli..31..189S. doi:10.1002/joc.2106. S2CID 54835415.
- ^ Chen, F.; Kusaka, H.; Bornstein, R.; Ching, J.; Grimmond, C. S. B.; Grossman-Clarke, S.; Loridan, T.; Manning, K. W.; Martilli, A.; Miao, S.; Sailor, D.; Salamanca, F. P.; Taha, H.; Tewari, M.; Wang, X.; Wyszogrodzki, A. A.; Zhang, C. (2011). "The integrated WRF/urban modelling system: Development, evaluation, and applications to urban environmental problems". International Journal of Climatology. 31 (2): 273. Bibcode:2011IJCli..31..273C. doi:10.1002/joc.2158. S2CID 54686199.
- ^ Union of Concerned Scientists. "Rising Temperatures, Worsening Ozone Pollution." Climate Change and Your Health (2011): n. pag. Print.
- ^ "Technical Summary". Climate Change 2022 – Impacts, Adaptation and Vulnerability. 2023. pp. 37–118. doi:10.1017/9781009325844.002. ISBN 978-1-009-32584-4.
- ^ Arizona Board of Regents (2006). "Urban Climate – Climate Study and UHI". Arizona State University. Archived from the original on 2007-11-23. Retrieved 2007-08-02.
- ^ Chiel C. van Heerwaarden & J. Vilà-Guerau de Arellano (2008). "Relative humidity as an indicator for cloud formation over heterogeneous land surfaces". Journal of the Atmospheric Sciences. 65 (10): 3263–3277. Bibcode:2008JAtS...65.3263V. doi:10.1175/2008JAS2591.1. S2CID 56010396.
- ^ Fuchs, Dale (2005-06-28). "Spain goes hi-tech to beat drought". The Guardian. Retrieved 2007-08-02.
- ^ Goddard Space Flight Center (2002-06-18). "NASA Satellite Confirms Urban Heat Islands Increase Rainfall Around Cities". National Aeronautics and Space Administration. Archived from the original on June 12, 2008. Retrieved 2009-07-17.
- ^ Zhou, Decheng; Zhao, Shuqing; Zhang, Liangxia; Sun, Ge; Liu, Yongqiang (10 June 2015). "The footprint of urban heat island effect in China". Scientific Reports. 5: 11160. Bibcode:2015NatSR...511160Z. doi:10.1038/srep11160. PMC 4461918. PMID 26060039.
- ^ Peterson, T.C.; Gallo, K.P.; Lawrimore, J.; Owen, T.W.; Huang, A.; McKittrick, D.A. (1999). "Global rural temperature trends". Geophysical Research Letters. 26 (3): 329–332. Bibcode:1999GeoRL..26..329P. doi:10.1029/1998GL900322.
- ^ J. Zhang, Guang; Cai, Ming; Hu, Aixue (27 January 2013). "Energy consumption and the unexplained winter warming over northern Asia and North America". Nature Climate Change. 3 (5): 466–470. Bibcode:2013NatCC...3..466Z. doi:10.1038/nclimate1803.
- ^ Broadbent, Ashley Mark; Krayenhoff, Eric Scott; Georgescu, Matei (13 August 2020). "The motley drivers of heat and cold exposure in 21st century US cities". Proceedings of the National Academy of Sciences. 117 (35): 21108–21117. Bibcode:2020PNAS..11721108B. doi:10.1073/pnas.2005492117. PMC 7474622. PMID 32817528.
- ^ J. F. Clarke (1972). "Some effects of the urban structure on heat mortality". Environmental Research. 5 (1): 93–104. Bibcode:1972ER......5...93C. doi:10.1016/0013-9351(72)90023-0. PMID 5032928.
- ^ Kovats, R. Sari; Hajat, Shakoor (April 2008). "Heat Stress and Public Health: A Critical Review". Annual Review of Public Health. 29 (1): 41–55. doi:10.1146/annurev.publhealth.29.020907.090843. PMID 18031221.
- ^ Adams-Fuller, Terri (2023-07-01). "Extreme Heat Is Deadlier Than Hurricanes, Floods and Tornadoes Combined". Scientific American. Retrieved 2024-05-02.
- ^ "The Impacts of Extreme Heat on Mental Health". Psychiatric Times. 2019-07-30. Retrieved 2024-05-02.
- ^ Cho, Hyunkuk (May 2017). "The effects of summer heat on academic achievement: A cohort analysis". Journal of Environmental Economics and Management. 83: 185–196. Bibcode:2017JEEM...83..185C. doi:10.1016/j.jeem.2017.03.005.
- ^ a b c d "Assessment of International Urban Heat Island Research" (PDF). U.S. Department of Energy Report. Navigant Consulting. Archived from the original (PDF) on 17 February 2013. Retrieved 30 April 2014.
- ^ a b Koppe, Christina; Sari Kovats; Gerd Jendritzky; Bettina Menne (2004). "Heat-waves: risks and responses". Health and Global Environmental Change Series. 2. Archived from the original on 2023-03-22. Retrieved 2014-05-07.
- ^ Shi, Yuan; Katzschner, Lutz; Ng, Edward (March 2018). "Modelling the fine-scale spatiotemporal pattern of urban heat island effect using land use regression approach in a megacity". Science of the Total Environment. 618: 891–904. Bibcode:2018ScTEn.618..891S. doi:10.1016/j.scitotenv.2017.08.252. PMID 29096959.
- ^ Wang, Pin; Goggins, William B.; Shi, Yuan; Zhang, Xuyi; Ren, Chao; Ka-Lun Lau, Kevin (June 2021). "Long-term association between urban air ventilation and mortality in Hong Kong". Environmental Research. 197: 111000. Bibcode:2021ER....19711000W. doi:10.1016/j.envres.2021.111000. PMID 33745928. S2CID 232310626.
- ^ Weng, Qihao; Firozjaei, Mohammad Karimi; Sedighi, Amir; Kiavarz, Majid; Alavipanah, Seyed Kazem (19 May 2019). "Statistical analysis of surface urban heat island intensity variations: A case study of Babol city, Iran". GIScience & Remote Sensing. 56 (4): 576–604. Bibcode:2019GISRS..56..576W. doi:10.1080/15481603.2018.1548080. S2CID 134003294.
- ^ Yuan, F (2007). "Comparison of impervious surface area and normalized difference vegetation index as indicators of surface urban heat island effects in Landsat imagery". Remote Sensing of Environment. 106 (3): 375–386. Bibcode:2007RSEnv.106..375Y. doi:10.1016/j.rse.2006.09.003.
- ^ NYS DEC. "Streams Tributary to Onondaga Lake Biological Assessment." Dec.ny.gov. N.p., 2008. Web. 12 September 2013.
- ^ "Fish Kill Event - McLoud Run". Iowa DNR Fish Kill Database.
- ^ Paul A. Tipler & Gene Mosca (2007). Physics for Scientists and Engineers. Macmillan. p. 686. ISBN 978-1-4292-0124-7.
- ^ "Urban Climate – Climate Study and UHI". United States Environmental Protection Agency. 2009-02-09. Retrieved 2009-06-18.
- ^ "Islands in the Sun". Institute on the Environment. University of Minnesota. Archived from the original on 2016-03-03. Retrieved 2014-11-11.
- ^ "Cool Pavement Report" (PDF). Environmental Protection Agency. June 2005. pp. 21, 43. Retrieved 2013-01-15.
- ^ a b c Shochat, Eyal; Warren, Paige S.; Faeth, Stanley H.; Mclntyre, Nancy E.; Hope, Diane (April 2006). "From Patterns to Emerging Processes in Mechanistic Urban Ecology". Trends in Ecology & Evolution. 21 (4): 186–91. doi:10.1016/j.tree.2005.11.019. PMID 16701084.
- ^ Tang, Teri (2014-06-05). "Where are the Insects?". School of Life Sciences. Arizona State University. Retrieved 19 October 2014.
- ^ Sheng-chieh Chang (2000-06-23). "Energy Use". Environmental Energies Technology Division. Archived from the original on March 11, 2009. Retrieved 2009-06-18.
- ^ "Aging and Weathering of Cool Roofing Membranes" (PDF). Cool Roofing Symposium. 2005-08-23. Archived from the original (PDF) on 2011-11-15. Retrieved 2010-08-16.
- ^ a b Rosenfeld, Arthur H.; Akbari, Hashem; Romm, Joseph J.; Pomerantz, Melvin (1998). "Cool communities: strategies for heat island mitigation and smog reduction" (PDF). Energy and Buildings. 28 (1): 51–62. Bibcode:1998EneBu..28...51R. doi:10.1016/S0378-7788(97)00063-7.
- ^ Salamanca, F.; Georgescu, M.; Mahalov, A.; Moustaoui, M.; Wang, M. (27 May 2014). "Anthropogenic heating of the urban environment due to air conditioning". Journal of Geophysical Research: Atmospheres. 119 (10): 5949–5965. Bibcode:2014JGRD..119.5949S. doi:10.1002/2013JD021225.
- ^ #author.fullName}. "Making roofs white or reflective is the best way to keep a city cool". New Scientist. Retrieved 2024-07-11.
{{cite web}}
:|last=
has generic name (help) - ^ Albers, R.A.W.; Bosch, P.R.; Blocken, B.; van den Dobbelsteen, A.A.J.F.; van Hove, L.W.A.; Spit, T.J.M.; van de Ven, F.; van Hooff, T.; Rovers, V. (January 2015). "Overview of challenges and achievements in the climate adaptation of cities and in the Climate Proof Cities program" (PDF). Building and Environment. 83: 1–10. Bibcode:2015BuEnv..83....1A. doi:10.1016/j.buildenv.2014.09.006. hdl:1874/309149.
- ^ Michał Kaszewski: „Miejska wyspa ciepła – sposoby jej ograniczania": Wykład popularno-naukowy: „Miejska wyspa ciepła – sposoby jej ograniczania"[unreliable source?]
- ^ a b Rosenfeld, Arthur H.; Romm, Joseph J.; Akbari, Hashem; Lloyd, Alan C. (February 1997). "Painting the town white—and green". Technology Review. 100 (2): 52–59.
- ^ "Top 22 Benefits of Trees". Tree People. Retrieved 7 July 2014.
- ^ "Trees and Vegetation". EPA.gov. 2014-02-28. Retrieved 7 July 2014.
- ^ a b c Zinzi, M.; Agnoli, S. (2012). "Cool and green roofs. An energy and comfort comparison between passive cooling and mitigation urban heat island techniques for residential buildings in the Mediterranean region". Energy and Buildings. 55: 66–76. Bibcode:2012EneBu..55...66Z. doi:10.1016/j.enbuild.2011.09.024.
- ^ #author.fullName}. "Making roofs white or reflective is the best way to keep a city cool". New Scientist. Retrieved 2024-07-11.
{{cite web}}
:|last=
has generic name (help) - ^ "Comprehensive Cool Roof Guide from the Vinyl Roofing Division of the Chemical Fabrics and Film Association". Archived from the original on 2013-09-21.
- ^ "Cool Pavement Report" (PDF). Environmental Protection Agency. June 2005. p. 14. Retrieved 2009-02-06.
- ^ Al Gore; A. Steffen (2008). World Changing: A User's Guide for the 21st Century. New York: Abrams. p. 258.
- ^ Yaghoobian, N.; Kleissl, J. (2012). "Effect of reflective pavements on building energy use". Urban Climate. 2: 25–42. Bibcode:2012UrbCl...2...25Y. doi:10.1016/j.uclim.2012.09.002.
- ^ Yang, Jiachuan; Wang, Zhihua; Kaloush, Kamil E. (October 2013), Unintended Consequences: A Research Synthesis Examining the Use of Reflective Pavements to Mitigate the Urban Heat Island Effect (PDF), Tempe, Arizona: NCE SMART Innovations, archived from the original (PDF) on 2013-12-02, retrieved 2013-11-25
- ^ "Whitest-ever paint could help cool heating Earth, study shows". The Guardian. April 15, 2021. Retrieved April 16, 2021.
- ^ Li, Xiangyu; Peoples, Joseph; Yao, Peiyan; Ruan, Xiulin (12 May 2021). "Ultrawhite BaSO 4 Paints and Films for Remarkable Daytime Subambient Radiative Cooling". ACS Applied Materials & Interfaces. 13 (18): 21733–21739. doi:10.1021/acsami.1c02368. PMID 33856776. S2CID 233259255.
- ^ "Green (Planted) Roofs". Archived from the original on 2011-07-28. Retrieved 2010-08-07.
- ^ Liu, Hongqing; Kong, Fanhua; Yin, Haiwei; Middel, Ariane; Zheng, Xiandi; Huang, Jing; Xu, Hairong; Wang, Ding; Wen, Zhihao (2021). "Impacts of green roofs on water, temperature, and air quality: A bibliometric review". Building and Environment. 196: 107794. Bibcode:2021BuEnv.19607794L. doi:10.1016/j.buildenv.2021.107794. ISSN 0360-1323.
- ^ "Four Barriers to Implementing Green Roofs – And How to Overcome Them | Nicole Holmes". Living Architecture Monitor. Retrieved 2024-07-01.
- ^ a b c Teotónio, Inês; Silva, Cristina Matos; Cruz, Carlos Oliveira (2021). "Economics of green roofs and green walls: A literature review". Sustainable Cities and Society. 69: 102781. Bibcode:2021SusCS..6902781T. doi:10.1016/j.scs.2021.102781. ISSN 2210-6707.
- ^ Feng, Haibo; Hewage, Kasun N. (2018). "Economic benefits and costs of green roofs". Nature based strategies for urban and building sustainability. Butterworth-Heinemann. pp. 307–318. doi:10.1016/B978-0-12-812150-4.00028-8. ISBN 978-0-12-812150-4.
- ^ https://www.gosnells.wa.gov.au/sites/default/files/seamless/3_stormwater_management.pdf
- ^ Wang, Junsong; Meng, Qinglin; Zou, Ya; Qi, Qianlong; Tan, Kanghao; Santamouris, Mat; He, Bao-Jie (August 2022). "Performance synergism of pervious pavement on stormwater management and urban heat island mitigation: A review of its benefits, key parameters, and co-benefits approach". Water Research. 221: 118755. Bibcode:2022WatRe.22118755W. doi:10.1016/j.watres.2022.118755. PMID 35728492.
- ^ Hiltrud Pötz & Pierre Bleuze (2011). Urban green-blue grids for sustainable and dynamic cities. Delft: Coop for life. ISBN 978-90-818804-0-4.
- ^ Chiesura, Anna (2004). "The role of urban parks for the sustainable city". Landscape and Urban Planning. 68 (1): 129–138. doi:10.1016/j.landurbplan.2003.08.003.
- ^ "Sustainable trade infrastructure in Africa: A key element for growth and prosperity?". International Centre for Trade and Sustainable Development.
- ^ "Nachhaltigesinvestment 2016". Archived from the original on 2017-01-23. Retrieved 2022-03-19.
- ^ Staddon, Chad; Ward, Sarah; De Vito, Laura; Zuniga-Teran, Adriana; Gerlak, Andrea K.; Schoeman, Yolandi; Hart, Aimee; Booth, Giles (September 2018). "Contributions of green infrastructure to enhancing urban resilience". Environment Systems and Decisions. 38 (3): 330–338. Bibcode:2018EnvSD..38..330S. doi:10.1007/s10669-018-9702-9. S2CID 62800263.
- ^ Heo, Se-Yeon; Lee, Gil Ju; Song, Young Min (2022). "Heat-shedding with photonic structures: radiative cooling and its potential". Journal of Materials Chemistry C. 10 (27): 9915–9937. doi:10.1039/D2TC00318J. S2CID 249695930.
- ^ Zhou, Kai; Miljkovic, Nenad; Cai, Lili (March 2021). "Performance analysis on system-level integration and operation of daytime radiative cooling technology for air-conditioning in buildings". Energy and Buildings. 235: 110749. Bibcode:2021EneBu.23510749Z. doi:10.1016/j.enbuild.2021.110749. S2CID 234180182.
- ^ Howard, Luke (2012) [1818]. The Climate of London Deduced from Meteorological Observations. Vol. 1. Cambridge University Press. ISBN 9781108049511.
- ^ Keith C. Heidorn (2009). "Luke Howard: The Man Who Named The Clouds". Islandnet.com. Retrieved 2009-06-18.
- ^ Stewart, Iain D. (December 2019). "Why should urban heat island researchers study history?". Urban Climate. 30: 100484. Bibcode:2019UrbCl..3000484S. doi:10.1016/j.uclim.2019.100484. S2CID 203337407.
- ^ Masson, Valéry; Lemonsu, Aude; Hidalgo, Julia; Voogt, James (2020-10-17). "Urban Climates and Climate Change". Annual Review of Environment and Resources. 45 (1): 411–444. doi:10.1146/annurev-environ-012320-083623.
- ^ Díaz, J.; Jordán, A.; García, R.; López, C.; Alberdi, J.; Hernández, E.; Otero, A. (1 February 2014). "Heat waves in Madrid 1986–1997: effects on the health of the elderly". International Archives of Occupational and Environmental Health. 75 (3): 163–170. doi:10.1007/s00420-001-0290-4. PMID 11954983. S2CID 31284700.
- ^ Harlan, Sharon L.; Brazel, Anthony J.; Prashad, Lela; Stefanov, William L.; Larsen, Larissa (December 2006). "Neighborhood microclimates and vulnerability to heat stress". Social Science & Medicine. 63 (11): 2847–2863. doi:10.1016/j.socscimed.2006.07.030. hdl:2286/R.I.55228. PMID 16996668.
- ^ US EPA, OAR (2019-11-06). "Heat Islands and Equity". www.epa.gov. Retrieved 2024-04-18.
- ^ Wilson, Bev (October 2020). "Urban Heat Management and the Legacy of Redlining". Journal of the American Planning Association. 86 (4): 443–457. doi:10.1080/01944363.2020.1759127.
- ^ Adams, Kaitlyn; Knuth, Colette Steward (January 2024). "The effect of urban heat islands on pediatric asthma exacerbation: How race plays a role". Urban Climate. 53: 101833. Bibcode:2024UrbCl..5301833A. doi:10.1016/j.uclim.2024.101833.
- ^ Zhu, Pengyu; Zhang, Yaoqi (March 2008). "Demand for urban forests in United States cities". Landscape and Urban Planning. 84 (3–4): 293–300. Bibcode:2008LUrbP..84..293Z. doi:10.1016/j.landurbplan.2007.09.005.
- ^ De Chant, Tim. "Urban Trees Reveal Income Inequality". Per Square Mile. Bibcode:2008LUrbP..84..293Z. doi:10.1016/j.landurbplan.2007.09.005. Retrieved 7 May 2014.
{{cite journal}}
: Cite journal requires|journal=
(help) - ^ Chant, Tim. "Urban Trees reveal income inequality". Per Square Mile. Bibcode:2008LUrbP..84..293Z. doi:10.1016/j.landurbplan.2007.09.005. Retrieved 7 July 2014.
- ^ DeSilver, Drew (2021-08-02). "As national eviction ban expires, a look at who rents and who owns in the U.S." Pew Research Center. Retrieved 2024-05-02.
- ^ Ramirez, Rachel (2022-07-20). "Faced with more deadly heat waves, US cities are taking an unprecedented step". CNN. Archived from the original on 2023-01-06. Retrieved 2023-01-06.
- ^ Moloney, Anastasia (2022-11-09). "How 'chief heat officers' keep cities cool as the world warms". Reuters. Archived from the original on 2023-01-06. Retrieved 2023-01-06.
- ^ Markey, Edward J. (2020-07-22). "S.4280 - 116th Congress (2019-2020): Preventing HEAT Illness and Deaths Act of 2020". www.congress.gov. Retrieved 2021-10-26.
- ^ "New federal bill supports heat island mitigation". U.S. Green Building Council. Retrieved 2021-10-26.
- ^ New York City Regional Heat Island Initiative (October 2006). "Mitigating New York City's Heat Island With Urban Forestry, Living Roofs, and Light Surfaces" (PDF). New York State Energy Research and Development Authority. p. ii. Retrieved 2009-06-18.[permanent dead link]
- ^ Wilmsen, Carl. Partnerships for Empowerment: Participatory Research for Community-based Natural Resource Management. London: Earthscan, 2008. Print.
- ^ "Cool solutions for urban heat islands | Stanford Woods Institute for the Environment". woods.stanford.edu. October 26, 2023. Retrieved 2024-05-02.
- ^ KristinaP. "Collaborative Heat Mapping Research Project". Virginia Foundation for Independent Colleges. Retrieved 2024-05-02.
- ^ "Cool Neighborhoods NYC". NYC Mayor's Office of Climate and Environmental Justice. 2017-06-14. Retrieved 2024-05-02.
External links
[edit]