Abiogenesis: Difference between revisions
Funandtrvl (talk | contribs) m rmv commentary from article |
Afterrock81 (talk | contribs) Undid revision 398388905 by Funandtrvl (talk) Here until redirects referring to the general origins of life are removed. |
||
Line 35: | Line 35: | ||
In 1768, [[Lazzaro Spallanzani]] demonstrated that [[microbe]]s were present in the air, and could be killed by boiling. In 1861, [[Louis Pasteur]] performed a series of experiments which demonstrated that organisms such as bacteria and fungi do not spontaneously appear in sterile, nutrient-rich media. |
In 1768, [[Lazzaro Spallanzani]] demonstrated that [[microbe]]s were present in the air, and could be killed by boiling. In 1861, [[Louis Pasteur]] performed a series of experiments which demonstrated that organisms such as bacteria and fungi do not spontaneously appear in sterile, nutrient-rich media. |
||
===Intelligent Design theory=== |
|||
According to Intelligent Design, there are too many individual factors involved with life on Earth that the probability of life spontaneously creating itself is close to 0 <ref>{{http://mathforum.org/dr.math/faq/faq.prob.intro.html}}</ref>. With an increase in specific variables necessary for life to exist on our planet, the number of factors involved reduces the probability. Therefore, it is mathematically impossible for our planet to sustain life with only chance. Our planet is very specifically placed within our solar system and galaxy with just the right factors allowing life on Earth to exist<ref>{{http://wiki.riteme.site/wiki/Rare_Earth_hypothesis}}</ref><ref>{{http://wiki.riteme.site/wiki/Planetary_habitability}}</ref>. Therefore, according to Intelligent Design life on Earth MUST have been created by an intelligence greater than ourselves. I trust you, the reader, has the intelligence needed to decide for yourself whether Intelligent Design has any basis for being an acceptable THEORY to how life on Earth began. |
|||
=== Pasteur and Darwin === |
=== Pasteur and Darwin === |
Revision as of 05:42, 23 November 2010
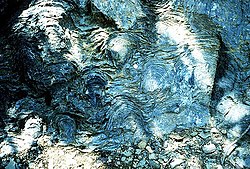
In natural science, abiogenesis (pronounced /ˌeɪbaɪ.ɵˈdʒɛnɨsɪs/, AY-bye-oh-JEN-ə-siss) or biopoesis is the study of how life arises from inanimate matter through natural processes, and the method by which life on Earth arose. Most amino acids, often called "the building blocks of life", can form via natural chemical reactions unrelated to life, as demonstrated in the Miller–Urey experiment and similar experiments, which involved simulating some of the conditions of the early Earth, in a scientific laboratory.[1] In all living things, these amino acids are organized into proteins, and the construction of these proteins is mediated by nucleic acids. Which of these organic molecules first arose and how they formed the first life is the focus of abiogenesis.
In any theory of abiogenesis, two aspects of life have to be accounted for: replication, and metabolism. The question of which came first gave rise to different types of theories. In the beginning, metabolism-first theories (Oparin coacervate) were proposed, and only later thinking gave rise to the modern, replication-first approach.
In modern, still somewhat limited understanding, the first living things on Earth are thought to be single cell prokaryotes (which lack a cell nucleus), perhaps evolved from protobionts (organic molecules surrounded by a membrane-like structure).[2] The oldest ancient fossil microbe-like objects are dated to be 3.5 Ga (billion years old), approximately one billion years after the formation of the Earth itself.[3][4] By 2.4 Ga, the ratio of stable isotopes of carbon, iron and sulfur shows the action of living things on inorganic minerals and sediments[5][6] and molecular biomarkers indicate photosynthesis, demonstrating that life on Earth was widespread by this time.[7][8]
The sequence of chemical events that led to the first nucleic acids is not known. Several hypotheses about early life have been proposed, most notably the iron-sulfur world theory (metabolism without genetics) and the RNA world hypothesis (RNA life-forms).
Conceptual history
Spontaneous generation
Until the early 19th century, people generally believed in the ongoing spontaneous generation of certain forms of life from non-living matter. This was paired with heterogenesis, the belief that one form of life derives from a different form (e.g. bees from flowers).[9] Classical notions of abiogenesis, now more precisely known as spontaneous generation, held that certain complex, living organisms are generated by decaying organic substances. According to Aristotle it was a readily observable truth that aphids arise from the dew which falls on plants, flies from putrid matter, mice from dirty hay, crocodiles from rotting logs at the bottom of bodies of water, and so on.[10]
In the 17th century, such assumptions started to be questioned; for example, in 1646, Sir Thomas Browne published his Pseudodoxia Epidemica (subtitled Enquiries into Very many Received Tenets, and Commonly Presumed Truths), which was an attack on false beliefs and "vulgar errors." His conclusions were not widely accepted. For example, his contemporary, Alexander Ross wrote: "To question this (i.e., spontaneous generation) is to question reason, sense and experience. If he doubts of this let him go to Egypt, and there he will find the fields swarming with mice, begot of the mud of Nylus, to the great calamity of the inhabitants."[11]
In 1665, Robert Hooke published the first drawings of a microorganism. Hooke was followed in 1676 by Anton van Leeuwenhoek, who drew and described microorganisms that are now thought to have been protozoa and bacteria.[12] Many felt the existence of microorganisms was evidence in support of spontaneous generation, since microorganisms seemed too simplistic for sexual reproduction, and asexual reproduction through cell division had not yet been observed.
The first solid evidence against spontaneous generation came in 1668 from Francesco Redi, who proved that no maggots appeared in meat when flies were prevented from laying eggs. It was gradually shown that, at least in the case of all the higher and readily visible organisms, the previous sentiment regarding spontaneous generation was false. The alternative seemed to be biogenesis: that every living thing came from a pre-existing living thing (omne vivum ex ovo, Latin for "every living thing from an egg").
In 1768, Lazzaro Spallanzani demonstrated that microbes were present in the air, and could be killed by boiling. In 1861, Louis Pasteur performed a series of experiments which demonstrated that organisms such as bacteria and fungi do not spontaneously appear in sterile, nutrient-rich media.
Intelligent Design theory
According to Intelligent Design, there are too many individual factors involved with life on Earth that the probability of life spontaneously creating itself is close to 0 [13]. With an increase in specific variables necessary for life to exist on our planet, the number of factors involved reduces the probability. Therefore, it is mathematically impossible for our planet to sustain life with only chance. Our planet is very specifically placed within our solar system and galaxy with just the right factors allowing life on Earth to exist[14][15]. Therefore, according to Intelligent Design life on Earth MUST have been created by an intelligence greater than ourselves. I trust you, the reader, has the intelligence needed to decide for yourself whether Intelligent Design has any basis for being an acceptable THEORY to how life on Earth began.
Pasteur and Darwin

By the middle of the 19th century, the theory of biogenesis had accumulated so much evidential support, due to the work of Louis Pasteur and others, that the alternative theory of spontaneous generation had been effectively disproven. Pasteur himself remarked, after a definitive finding in 1864, "Never will the doctrine of spontaneous generation recover from the mortal blow struck by this simple experiment."[16] The collapse of spontaneous generation, however, left a vacuum of scientific thought on the question of how life had first arisen.
In a letter to Joseph Dalton Hooker on February 1, 1871,[17] Charles Darwin addressed the question, suggesting that the original spark of life may have begun in a "warm little pond, with all sorts of ammonia and phosphoric salts, lights, heat, electricity, etc. present, so that a protein compound was chemically formed ready to undergo still more complex changes". He went on to explain that "at the present day such matter would be instantly devoured or absorbed, which would not have been the case before living creatures were formed."[18] In other words, the presence of life itself makes the search for the origin of life dependent on the sterile conditions of the laboratory.
"Primordial soup" theory
No new notable research or theory on the subject appeared until 1924, when Alexander Oparin reasoned that atmospheric oxygen prevents the synthesis of certain organic compounds that are necessary building blocks for the evolution of life. In his The Origin of Life,[19][20] Oparin proposed that the "spontaneous generation of life" that had been attacked by Louis Pasteur, did in fact occur once, but was now impossible because the conditions found in the early earth had changed, and the presence of living organisms would immediately consume any spontaneously generated organism. Oparin argued that a "primeval soup" of organic molecules could be created in an oxygen-less atmosphere through the action of sunlight. These would combine in ever-more complex fashions until they formed coacervate droplets. These droplets would "grow" by fusion with other droplets, and "reproduce" through fission into daughter droplets, and so have a primitive metabolism in which those factors which promote "cell integrity" survive, and those that do not become extinct. Many modern theories of the origin of life still take Oparin's ideas as a starting point.
Around the same time, J. B. S. Haldane suggested that the Earth's pre-biotic oceans–very different from their modern counterparts–would have formed a "hot dilute soup" in which organic compounds could have formed. This idea was called biopoiesis or biopoesis, the process of living matter evolving from self-replicating but nonliving molecules.[21][22]
Early conditions
Morse and MacKenzie have suggested that oceans may have appeared first in the Hadean eon, as soon as two hundred million years (200 Ma) after the Earth was formed, in a hot 100 °C (212 °F) reducing environment, and that the pH of about 5.8 rose rapidly towards neutral.[23] This has been supported by Wilde[3] who has pushed the date of the zircon crystals found in the metamorphosed quartzite of Mount Narryer in Western Australia, previously thought to be 4.1–4.2 Ga, to 4.404 Ga. This means that oceans and continental crust existed within 150 Ma of Earth's formation.
Despite this, the Hadean environment was one highly hazardous to life. Frequent collisions with large objects, up to 500 kilometres (310 mi) in diameter, would have been sufficient to vaporise the ocean within a few months of impact, with hot steam mixed with rock vapour leading to high altitude clouds completely covering the planet. After a few months the height of these clouds would have begun to decrease but the cloud base would still have been elevated for about the next thousand years. After that, it would have begun to rain at low altitude. For another two thousand years rains would slowly have drawn down the height of the clouds, returning the oceans to their original depth only 3,000 years after the impact event.[24]
Between 3.8 and 4.1 Ga, changes in the orbits of the gaseous giant planets may have caused a late heavy bombardment that pockmarked the moon and other inner planets (Mercury, Mars, and presumably Earth and Venus). This would likely have sterilized the planet had life appeared before that time.
By examining the time interval between such devastating environmental events, the time interval when life might first have come into existence can be found for different early environments. The study by Maher and Stevenson shows that if the deep marine hydrothermal setting provides a suitable site for the origin of life, abiogenesis could have happened as early as 4.0 to 4.2 Ga, whereas if it occurred at the surface of the earth abiogenesis could only have occurred between 3.7 and 4.0 Ga.[25]
Other research suggests a colder start to life. Work by Leslie Orgel and colleagues on the synthesis of purines has shown that freezing temperatures are advantageous, due to the concentrating effect for key precursors such as hydrogen cyanide.[26] Research by Stanley Miller and colleagues suggested that while adenine and guanine require freezing conditions for synthesis, cytosine and uracil may require boiling temperatures.[27] Based on this research, Miller suggested a beginning of life involving freezing conditions and exploding meteorites.[28] An article in Discover Magazine points to research by the Miller group indicating the formation of seven different amino acids and 11 types of nucleobases in ice when ammonia and cyanide were left in a freezer from 1972–1997.[29][30] This article also describes research by Christof Biebricher showing the formation of RNA molecules 400 bases long under freezing conditions using an RNA template, a single-strand chain of RNA that guides the formation of a new strand of RNA. As that new RNA strand grows, it adheres to the template.[31] The explanation given for the unusual speed of these reactions at such a low temperature is eutectic freezing. As an ice crystal forms, it stays pure: only molecules of water join the growing crystal, while impurities like salt or cyanide are excluded. These impurities become crowded in microscopic pockets of liquid within the ice, and this crowding causes the molecules to collide more often.
Evidence of the early appearance of life comes from the Isua supercrustal belt in Western Greenland and from similar formations in the nearby Akilia Islands. Carbon entering into rock formations has a ratio of Carbon-13 (13C) to Carbon-12 (12C) of about −5.5 (in units of δ13C), where because of a preferential biotic uptake of 12C, biomass has a δ13C of between −20 and −30. These isotopic fingerprints are preserved in the sediments, and Mojzis has used this technique to suggest that life existed on the planet already by 3.85 billion years ago.[32] Lazcano and Miller (1994) suggest that the rapidity of the evolution of life is dictated by the rate of recirculating water through mid-ocean submarine vents. Complete recirculation takes 10 million years, thus any organic compounds produced by then would be altered or destroyed by temperatures exceeding 300 °C (572 °F). They estimate that the development of a 100 kilobase genome of a DNA/protein primitive heterotroph into a 7000 gene filamentous cyanobacterium would have required only 7 Ma.[33]
Current models
There is no truly "standard model" of the origin of life. Most currently accepted models draw at least some elements from the framework laid out by the Oparin-Haldane hypothesis. Under that umbrella, however, are a wide array of disparate discoveries and conjectures such as the following, listed in a rough order of postulated emergence:
- Some theorists suggest that the atmosphere of the early Earth may have been chemically reducing in nature, composed primarily of methane (CH4), ammonia (NH3), water (H2O), hydrogen sulfide (H2S), carbon dioxide (CO2) or carbon monoxide (CO), and phosphate (PO43-), with molecular oxygen (O2) and ozone (O3) either rare or absent.
- In such a reducing atmosphere, electrical activity can catalyze the creation of certain basic small molecules (monomers) of life, such as amino acids. This was demonstrated in the Miller–Urey experiment by Stanley L. Miller and Harold C. Urey in 1953.
- Phospholipids (of an appropriate length) can spontaneously form lipid bilayers, a basic component of the cell membrane.
- A fundamental question is about the nature of the first self-replicating molecule. Since replication is accomplished in modern cells through the cooperative action of proteins and nucleic acids, the major schools of thought about how the process originated can be broadly classified as "proteins first" and "nucleic acids first".
- The principal thrust of the "nucleic acids first" argument is as follows:
- The polymerization of nucleotides into random RNA molecules might have resulted in self-replicating ribozymes (RNA world hypothesis)
- Selection pressures for catalytic efficiency and diversity might have resulted in ribozymes which catalyse peptidyl transfer (hence formation of small proteins), since oligopeptides complex with RNA to form better catalysts. The first ribosome might have been created by such a process, resulting in more prevalent protein synthesis.
- Synthesized proteins might then outcompete ribozymes in catalytic ability, and therefore become the dominant biopolymer, relegating nucleic acids to their modern use, predominantly as a carrier of genomic information.
As of 2010, no one has yet synthesized a "protocell" using basic components which would have the necessary properties of life (the so-called "bottom-up-approach"). Without such a proof-of-principle, explanations have tended to be short on specifics. However, some researchers are working in this field, notably Steen Rasmussen at Los Alamos National Laboratory and Jack Szostak at Harvard University. Others have argued that a "top-down approach" is more feasible. One such approach, successfully attempted by Craig Venter and others at The Institute for Genomic Research, involves engineering existing prokaryotic cells with progressively fewer genes, attempting to discern at which point the most minimal requirements for life were reached.[34][35] The biologist John Desmond Bernal coined the term Biopoesis for this process [citation needed], and suggested that there were a number of clearly defined "stages" that could be recognised in explaining the origin of life.
- Stage 1: The origin of biological monomers
- Stage 2: The origin of biological polymers
- Stage 3: The evolution from molecules to cell
Bernal suggested that evolution may have commenced early, some time between Stage 1 and 2.[36]
Origin of organic molecules
There are two possible sources of organic molecules on the early Earth:
- Terrestrial origins–organic synthesis driven by impact shocks or by other energy sources (such as ultraviolet light or electrical discharges) (eg.Miller's experiments)
- Extraterrestrial origins–delivery by objects (e.g. carbonaceous chondrites) or gravitational attraction of organic molecules or primitive life-forms from space
Recently, estimates of these sources suggest that the heavy bombardment before 3.5 Ga within the early atmosphere made available quantities of organics comparable to those produced by other energy sources.[37]
"Soup" theory today: Miller's experiment and subsequent work
Biochemist Robert Shapiro has summarized the "Primordial Soup" theory of Oparin and Haldane in its "mature form" as follows:[38]
- The early Earth had a chemically reducing atmosphere.
- This atmosphere, exposed to energy in various forms, produced simple organic compounds ("monomers").
- These compounds accumulated in a "soup", which may have been concentrated at various locations (Shorelines, oceanic vents etc.).
- By further transformation, more complex organic polymers— and ultimately life— developed in the soup.
Regarding the reducing atmosphere
Whether the mixture of gases used in the Miller–Urey experiment truly reflects the atmospheric content of early Earth is a controversial topic. Other less reducing gases produce a lower yield and variety. It was once thought that appreciable amounts of molecular oxygen were present in the prebiotic atmosphere [citation needed], which would have essentially prevented the formation of organic molecules; however, the current scientific consensus is that such was not the case. (See Oxygen catastrophe).
Regarding monomer formation
One of the most important pieces of experimental support for the "soup" theory came in 1953. A graduate student, Stanley Miller, and his professor, Harold Urey, performed an experiment that demonstrated how organic molecules could have spontaneously formed from inorganic precursors, under conditions like those posited by the Oparin-Haldane Hypothesis. The now-famous "Miller–Urey experiment" used a highly reduced mixture of gases–methane, ammonia and hydrogen–to form basic organic monomers, such as amino acids.[39] This provided direct experimental support for the second point of the "soup" theory, and it is around the remaining two points of the theory that much of the debate now centers.
Apart from the Miller–Urey experiment, the next most important step in research on prebiotic organic synthesis was the demonstration by Joan Oró that the nucleic acid purine base, adenine, was formed by heating aqueous ammonium cyanide solutions.[40] In support of abiogenesis in eutectic ice, more recent work demonstrated the formation of s-triazines (alternative nucleobases), pyrimidines (including cytosine and uracil), and adenine from urea solutions subjected to freeze-thaw cycles under a reductive atmosphere (with spark discharges as an energy source).[41]
Regarding monomer accumulation
The "soup" theory relies on the assumption proposed by Darwin that in an environment with no pre-existing life, organic molecules may have accumulated and provided an environment for chemical evolution.
Regarding further transformation
The spontaneous formation of complex polymers from abiotically generated monomers under the conditions posited by the "soup" theory is not at all a straightforward process. Besides the necessary basic organic monomers, compounds that would have prohibited the formation of polymers were formed in high concentration during the Miller–Urey and Oró experiments. The Miller experiment, for example, produces many substances that would undergo cross-reactions with the amino acids or terminate the peptide chain.
More fundamentally, it can be argued that the most crucial challenge unanswered by this theory is how the relatively simple organic building blocks polymerise and form more complex structures, interacting in consistent ways to form a protocell. For example, in an aqueous environment hydrolysis of oligomers/polymers into their constituent monomers would be favored over the condensation of individual monomers into polymers.
The deep sea vent theory
The deep sea vent, or hydrothermal vent, theory for the origin of life on Earth posits that life may have begun at submarine hydrothermal vents, where hydrogen-rich fluids emerge from below the sea floor and interface with carbon dioxide-rich ocean water. Sustained chemical energy in such systems is derived from redox reactions, in which electron donors, such as molecular hydrogen, react with electron acceptors, such as carbon dioxide (see iron-sulfur world theory).
Fox's experiments
In the 1950s and 1960s, Sidney W. Fox studied the spontaneous formation of peptide structures under conditions that might plausibly have existed early in Earth's history. He demonstrated that amino acids could spontaneously form small peptides. These amino acids and small peptides could be encouraged to form closed spherical membranes, called protenoid microspheres, which show many of the basic characteristics of 'life'.[42]
Eigen's hypothesis
In the early 1970s the problem of the origin of life was approached by Manfred Eigen and Peter Schuster of the Max Planck Institute for Biophysical Chemistry. They examined the transient stages between the molecular chaos and a self-replicating hypercycle in a prebiotic soup.[43]
In a hypercycle, the information storing system (possibly RNA) produces an enzyme, which catalyzes the formation of another information system, in sequence until the product of the last aids in the formation of the first information system. Mathematically treated, hypercycles could create quasispecies, which through natural selection entered into a form of Darwinian evolution. A boost to hypercycle theory was the discovery that RNA, in certain circumstances, forms itself into ribozymes, capable of catalyzing their own chemical reactions.[44] However, these reactions are limited to self-excisions (in which a longer RNA molecule becomes shorter), and much rarer small additions that are incapable of coding for any useful protein. The hypercycle theory is further degraded since the hypothetical RNA would require the existence of complex biochemicals such as nucleotides which are not formed under the conditions proposed by the Miller–Urey experiment.
Hoffmann's contributions
Geoffrey W. Hoffmann, a student of Eigen, contributed to the concept of life involving both replication and metabolism emerging from catalytic noise. His contributions included showing that an early sloppy translation machinery can be stable against an error catastrophe of the type that had been envisaged as problematical by Leslie Orgel ("Orgel's paradox")[45][46] and calculations regarding the occurrence of a set of required catalytic activities together with the exclusion of catalytic activities that would be disruptive. This is called the stochastic theory of the origin of life.[47]
Wächtershäuser's hypothesis

Another possible answer to this polymerization conundrum was provided in 1980s by the German chemist Günter Wächtershäuser, in his iron-sulfur world theory. In this theory, he postulated the evolution of (bio)chemical pathways as fundamentals of the evolution of life. Moreover, he presented a consistent system of tracing today's biochemistry back to ancestral reactions that provide alternative pathways to the synthesis of organic building blocks from simple gaseous compounds.
In contrast to the classical Miller experiments, which depend on external sources of energy (such as simulated lightning or UV irradiation), "Wächtershäuser systems" come with a built-in source of energy, sulfides of iron and other minerals (e.g. pyrite). The energy released from redox reactions of these metal sulfides is not only available for the synthesis of organic molecules, but also for the formation of oligomers and polymers. It is therefore hypothesized that such systems may be able to evolve into autocatalytic sets of self-replicating, metabolically active entities that would predate the life forms known today.
The experiment produced a relatively small yield of dipeptides (0.4% to 12.4%) and a smaller yield of tripeptides (0.10%) but the authors also noted that: "under these same conditions dipeptides hydrolysed rapidly."[48]
Radioactive beach hypothesis
Zachary Adam at the University of Washington, Seattle, claims that stronger tidal processes from a much closer moon may have concentrated grains of uranium and other radioactive elements at the high water mark on primordial beaches where they may have been responsible for generating life's building blocks.[49] According to computer models reported in Astrobiology,[50] a deposit of such radioactive materials could show the same self-sustaining nuclear reaction as that found in the Oklo uranium ore seam in Gabon. Such radioactive beach sand provides sufficient energy to generate organic molecules, such as amino acids and sugars from acetonitrile in water. Radioactive monazite also releases soluble phosphate into regions between sand-grains, making it biologically "accessible". Thus amino acids, sugars and soluble phosphates can all be simultaneously produced, according to Adam. Radioactive actinides, then in greater concentrations, could have formed part of organo-metallic complexes. These complexes could have been important early catalysts to living processes.
John Parnell of the University of Aberdeen suggests that such a process could provide part of the "crucible of life" on any early wet rocky planet, so long as the planet is large enough to have generated a system of plate tectonics which brings radioactive minerals to the surface. As the early Earth is believed to have had many smaller "platelets" it would provide a suitable environment for such processes.[51]
Thermodynamic Origin of Life: Ultraviolet and Temperature-Assisted Replication (UVTAR) Model
Karo Michaelian of the National Autonomous University of Mexico (UNAM) points out that any model for the origin of life must take into account the fact that life is an irreversible thermodynamic process which arises and persists to produce entropy. Entropy production is not incidental to the process of life, but rather the fundamental reason for its existence. Present day life augments the entropy production of Earth by catalysing the water cycle through evapotranspiration.[52] Michaelian argues that if the thermodynamic function of life today is to produce entropy through coupling with the water cycle, then this probably was its function at its very beginnings. It turns out that both RNA and DNA when in water solution are very strong absorbers and extremely rapid dissipaters of ultraviolet light within the 200 nm - 300 nm wavelength range, just that high energy part of the sun's spectrum that could have penetrated the dense prebiotic atmosphere. Cnossen et al.[53] have shown that the amount of UV light reaching the Earth's surface in the Archean could have been up to 31 orders of magnitude larger than it is today at 260 nm where RNA and DNA absorb most strongly. Absorption and dissipation of UV light by these organic molecules at the Archean ocean surface would have increased significantly the temperature of the surface skin layer leading to enhanced evaporation and thus augmenting the primitive water cycle. Since absorption and dissipation of high energy photons is an entropy producing process, Michaelian argues that non-equilbrium abiogenic synthesis of RNA and DNA utilizing UV light [54] would have been thermodynamically favored.
A simple mechanism to explain the replication of RNA and DNA without the use of enzymes can also be given within the same thermodynamic framework by assuming that life arose when the temperature of the primitive seas had cooled to somewhat below the denaturing temperature of RNA or DNA (based on the ratio of 18O/16O found in cherts of the Barberton greenstone belt of South Africa of about 3.5 to 3.2 Ga., surface temperatures are predicted to have been around 70±15 °C,[55] similar to RNA or DNA denaturing temperatures). During the night, the surface water temperature would be below the denaturing temperature and single strand RNA/DNA could act as a template for the formation of double strand RNA/DNA. During the daylight hours, RNA and DNA would absorb UV light and convert this directly to heating of the ocean surface, raising the local temperature enough to allow for denaturing of RNA and DNA. The copying process would be repeated during the cool period overnight.[56] Such a temperature assisted mechanism of replication bears similarity to Polymerase Chain Reaction (PCR), a routine laboratory procedure to multiply DNA segments. Michaelian suggests that traditional origin of life research, expecting to describe the emergence of life from near-equilibrium conditions, is erroneous and that non-equilibrium conditions must be considered, in particular, the importance of entropy production to the emergence of life.
Since denaturation would be most probable in the late afternoon when the Archean sea surface temperature would be highest, and since late afternoon submarine sunlight is somewhat circularly polarized, the homochirality of the organic molecules of life can also be explained within the proposed thermodynamic framework.[57] [58]
Models to explain homochirality
Some process in chemical evolution must account for the origin of homochirality, i.e. all building blocks in living organisms having the same "handedness" (amino acids being left-handed, nucleic acid sugars (ribose and deoxyribose) being right-handed, and chiral phosphoglycerides). Chiral molecules can be synthesized, but in the absence of a chiral source or a chiral catalyst, they are formed in a 50/50 mixture of both enantiomers. This is called a racemic mixture. Clark has suggested that homochirality may have started in space, as the studies of the amino acids on the Murchison meteorite showed L-alanine to be more than twice as frequent as its D form, and L-glutamic acid was more than 3 times prevalent than its D counterpart. It is suggested that polarised light has the power to destroy one enantiomer within the proto-planetary disk. Noyes[59] showed that beta decay caused the breakdown of D-leucine, in a racemic mixture, and that the presence of 14C, present in larger amounts in organic chemicals in the early Earth environment, could have been the cause. Robert M. Hazen reports upon experiments conducted in which various chiral crystal surfaces act as sites for possible concentration and assembly of chiral monomer units into macromolecules.[60] Once established, chirality would be selected for.[61] Work with organic compounds found on meteorites tends to suggest that chirality is a characteristic of abiogenic synthesis, as amino acids show a left-handed bias, whereas sugars show a predominantly right-handed bias.[62]
Self-organization and replication
While features of self-organization and self-replication are often considered the hallmark of living systems, there are many instances of abiotic molecules exhibiting such characteristics under proper conditions. For example Martin and Russel show that physical compartmentation by cell membranes from the environment and self-organization of self-contained redox reactions are the most conserved attributes of living things, and they argue therefore that inorganic matter with such attributes would be life's most likely last common ancestor.[63]
Virus self-assembly within host cells has implications for the study of the origin of life,[64] as it lends further credence to the hypothesis that life could have started as self-assembling organic molecules.[65] [66]
From organic molecules to protocells
The question "How do simple organic molecules form a protocell?" is largely unanswered but there are many hypotheses. Some of these postulate the early appearance of nucleic acids ("genes-first") whereas others postulate the evolution of biochemical reactions and pathways first ("metabolism-first"). Recently, trends are emerging to create hybrid models that combine aspects of both.
"Genes first" models: the RNA world
The RNA world hypothesis describes an early Earth with self-replicating and catalytic RNA but no DNA or proteins. This has spurred scientists to try to determine if relatively short RNA molecules could have spontaneously formed that were capable of catalyzing their own continuing replication.[67] A number of hypotheses of modes of formation have been put forward. Early cell membranes could have formed spontaneously from proteinoids, protein-like molecules that are produced when amino acid solutions are heated–when present at the correct concentration in aqueous solution, these form microspheres which are observed to behave similarly to membrane-enclosed compartments. Other possibilities include systems of chemical reactions taking place within clay substrates or on the surface of pyrite rocks. Factors supportive of an important role for RNA in early life include its ability to act both to store information and catalyse chemical reactions (as a ribozyme); its many important roles as an intermediate in the expression and maintenance of the genetic information (in the form of DNA) in modern organisms; and the ease of chemical synthesis of at least the components of the molecule under conditions approximating the early Earth. Relatively short RNA molecules which can duplicate others have been artificially produced in the lab.[68] Such replicase RNA, which functions as both code and catalyst provides a template upon which copying can occur. Jack Szostak has shown that certain catalytic RNAs can, indeed, join smaller RNA sequences together, creating the potential, in the right conditions for self-replication. If these were present, Darwinian selection would favour the proliferation of such self-catalysing structures, to which further functionalities could be added.[69] Lincoln and Joyce identified an RNA enzyme capable of self sustained replication.[70]
Researchers have pointed out difficulties for the abiotic synthesis of nucleotides from cytosine and uracil.[71] Cytosine has a half-life of 19 days at 100 °C (212 °F) and 17,000 years in freezing water.[72] Larralde et al., say that "the generally accepted prebiotic synthesis of ribose, the formose reaction, yields numerous sugars without any selectivity."[73] and they conclude that their "results suggest that the backbone of the first genetic material could not have contained ribose or other sugars because of their instability." The ester linkage of ribose and phosphoric acid in RNA is known to be prone to hydrolysis.[74]
A slightly different version of the RNA-world hypothesis is that a different type of nucleic acid, such as PNA, TNA or GNA, was the first one to emerge as a self-reproducing molecule, to be replaced by RNA only later.[75][76] Pyrimidine ribonucleosides and their respective nucleotides have been prebiotically synthesised by a sequence of reactions which by-pass the free sugars, and are assembled in a stepwise fashion by going against the dogma that nitrogenous and oxygenous chemistries should be avoided. In a series of publications, The Sutherland Group at the School of Chemistry, University of Manchester have demonstrated high yielding routes to cytidine and uridine ribonucleotides built from small 2 and 3 carbon fragments such as glycolaldehyde, glyceraldehyde or glyceraldehyde-3-phosphate, cyanamide and cyanoacetylene. One of the steps in this sequence allows the isolation of enantiopure ribose aminooxazoline if the enantiomeric excess of glyceraldehyde is 60 % or greater.[77] This can be viewed as a prebiotic purification step, where the said compound spontaneously crystallised out from a mixture of the other pentose aminooxazolines. Ribose aminooxazoline can then react with cyanoacetylene in a mild and highly efficient manner to give the alpha cytidine ribonucleotide. Photoanomerization with UV light allows for inversion about the 1' anomeric centre to give the correct beta stereochemistry.[78] In 2009 they showed that the same simple building blocks allow access, via phosphate controlled nucleobase elaboration, to 2',3'-cyclic pyrimidine nucleotides directly, which are known to be able to polymerise into RNA. This paper also highlights the possibility for the photo-sanitization of the pyrimidine-2',3'-cyclic phosphates.[54] James Ferris's studies have shown that clay minerals of montmorillonite will catalyze the formation of RNA in aqueous solution, by joining activated mono RNA nucleotides to join together to form longer chains.[79] Although these chains have random sequences, the possibility that one sequence began to non-randomly increase its frequency by increasing the speed of its catalysis is possible to "kick start" biochemical evolution.
"Metabolism first" models
Several models reject the idea of the self-replication of a "naked-gene" and postulate the emergence of a primitive metabolism which could provide an environment for the later emergence of RNA replication.
Iron-sulfur world
One of the earliest incarnations of this idea was put forward in 1924 with Alexander Oparin's notion of primitive self-replicating vesicles which predated the discovery of the structure of DNA. More recent variants in the 1980s and 1990s include Günter Wächtershäuser's iron-sulfur world theory and models introduced by Christian de Duve based on the chemistry of thioesters. More abstract and theoretical arguments for the plausibility of the emergence of metabolism without the presence of genes include a mathematical model introduced by Freeman Dyson in the early 1980s and Stuart Kauffman's notion of collectively autocatalytic sets, discussed later in that decade.
However, the idea that a closed metabolic cycle, such as the reductive citric acid cycle, could form spontaneously (proposed by Günter Wächtershäuser) remains debated. In an article entitled "Self-Organizing Biochemical Cycles",[80] the late Leslie Orgel summarized his analysis of the proposal by stating, "There is at present no reason to expect that multistep cycles such as the reductive citric acid cycle will self-organize on the surface of FeS/FeS2 or some other mineral." It is possible that another type of metabolic pathway was used at the beginning of life. For example, instead of the reductive citric acid cycle, the "open" acetyl-CoA pathway (another one of the five recognised ways of carbon dioxide fixation in nature today) would be compatible with the idea of self-organisation on a metal sulfide surface. The key enzyme of this pathway, carbon monoxide dehydrogenase/acetyl-CoA synthase harbours mixed nickel-iron-sulfur clusters in its reaction centers and catalyses the formation of acetyl-CoA (which may be regarded as a modern form of acetyl-thiol) in a single step.
Thermosynthesis world
Today’s bioenergetic process of fermentation is related to the just mentioned citric acid cycle or the Acetyl-CoA pathway that have been connected to the primordial iron-sulfur world. In a different approach, today’s bioenergetic process of chemiosmosis, which plays an essential role in cellular respiration and photosynthesis, is considered as more fundamental than fermentation: in Anthonie Muller’s “thermosynthesis world” the ATP Synthase enzyme that sustains chemiosmosis is proposed as today’s enzyme that is the closest connected to the first metabolic process.[81][82]
First life needed an energy source to bring about the condensation reaction that yielded the peptide bonds of proteins and the phosphodiester bonds of RNA. In a generalization and thermal variation of the binding change mechanism of today’s ATP Synthase, the “First Protein” would have bound substrates (peptides, phosphate, nucleosides, RNA ‘monomers’) and condensed them to a reaction product that remained bound until it after a temperature change was released upon a thermal unfolding.
The energy source of the thermosynthesis world was thermal cycling, the result of suspension of the protocell in a convection current, as is plausible in a volcanic hot spring; the convection accounts for the self-organization and dissipative structure required in any origin of life model. The still ubiquitous role of thermal cycling in germination and cell division is considered a relic of primordial thermosynthesis.
By phosphorylating cell membrane lipids, this ‘First Protein’ gave a selective advantage to the lipid protocell that contained the protein. In the beginning this First Protein also synthesized a library with many proteins, of which only a minute fraction had thermosynthesis capabilities. Just as proposed by Dyson [83] for the first proteins, the First Protein propagated functionally: it made daughters with similar capabilities, but it did not copy itself. Functioning daughters consisted of different amino acid sequences.
Over a long time, RNA sequences were selected among the at first randomly synthesized RNAs by the criterion of speed and efficiency increase of First Protein synthesis, for instance by the creation of RNA that functioned as messenger RNA,[84] Transfer RNA[85] and ribosomal RNA, or, even more generally, all the components of the RNA World were also generated and selected. The thermosynthesis world therefore in theory accounts for the origin of the genetic machinery.
Whereas the iron-sulfur world identifies a circular pathway as the most simple—and therefore assumes the existence of enzymes—the thermosynthesis world does not even invoke a pathway, and does not assume the existence of regular enzymes: ATP Synthase’s binding change mechanism resembles a physical adsorption process that yields free energy,[86] rather than a regular enzyme’s mechanism, which decreases the free energy. The RNA World also implies the existence of several enzymes. But even the emergence of a single enzyme by chance is implausible.[87] The thermosynthesis world is therefore more simple, and thus more plausible, than the iron-sulfur and RNA worlds.
Possible role of bubbles
Waves breaking on the shore create a delicate foam composed of bubbles. Winds sweeping across the ocean have a tendency to drive things to shore, much like driftwood collecting on the beach. It is possible that organic molecules were concentrated on the shorelines in much the same way. Shallow coastal waters also tend to be warmer, further concentrating the molecules through evaporation. While bubbles composed mostly of water burst quickly, water containing amphiphiles forms much more stable bubbles, lending more time to the particular bubble to perform these crucial reactions.
Amphiphiles are oily compounds containing a hydrophilic head on one or both ends of a hydrophobic molecule. Some amphiphiles have the tendency to spontaneously form membranes in water. A spherically closed membrane contains water and is a hypothetical precursor to the modern cell membrane. If a protein would increase the integrity of its parent bubble, that bubble had an advantage, and was placed at the top of the natural selection waiting list. Primitive reproduction can be envisioned when the bubbles burst, releasing the results of the 'experiment' into the surrounding medium. Once enough of the 'right stuff' was released into the medium, the development of the first prokaryotes, eukaryotes, and multicellular organisms could be achieved.[88]
Similarly, bubbles formed entirely out of protein-like molecules, called microspheres, will form spontaneously under the right conditions. But they are not a likely precursor to the modern cell membrane, as cell membranes are composed primarily of lipid compounds rather than amino-acid compounds (for types of membrane spheres associated with abiogenesis, see protobionts, micelle, coacervate).
A recent model by Fernando and Rowe[89] suggests that the enclosure of an autocatalytic non-enzymatic metabolism within protocells may have been one way of avoiding the side-reaction problem that is typical of metabolism first models.
Other models
Autocatalysis
In 1993 Stuart Kauffman proposed that life initially arose as autocatalytic chemical networks.[90]
British ethologist Richard Dawkins wrote about autocatalysis as a potential explanation for the origin of life in his 2004 book The Ancestor's Tale. Autocatalysts are substances which catalyze the production of themselves, and therefore have the property of being a simple molecular replicator. In his book, Dawkins cites experiments performed by Julius Rebek and his colleagues at the Scripps Research Institute in California in which they combined amino adenosine and pentafluorophenyl ester with the autocatalyst amino adenosine triacid ester (AATE). One system from the experiment contained variants of AATE which catalysed the synthesis of themselves. This experiment demonstrated the possibility that autocatalysts could exhibit competition within a population of entities with heredity, which could be interpreted as a rudimentary form of natural selection.
Clay theory
A model for the origin of life based on clay was forwarded by A. Graham Cairns-Smith of the University of Glasgow in 1985 and explored as a plausible illustration by several other scientists, including Richard Dawkins.[91] Clay theory postulates that complex organic molecules arose gradually on a pre-existing, non-organic replication platform—silicate crystals in solution. Complexity in companion molecules developed as a function of selection pressures on types of clay crystal is then exapted to serve the replication of organic molecules independently of their silicate "launch stage".
Cairns-Smith is a staunch critic of other models of chemical evolution.[92] However, he admits that like many models of the origin of life, his own also has its shortcomings (Horgan 1991).
In 2007, Kahr and colleagues reported their experiments to examine the idea that crystals can act as a source of transferable information, using crystals of potassium hydrogen phthalate. "Mother" crystals with imperfections were cleaved and used as seeds to grow "daughter" crystals from solution. They then examined the distribution of imperfections in the crystal system and found that the imperfections in the mother crystals were indeed reproduced in the daughters. The daughter crystals had many additional imperfections. For a gene-like behavior the additional imperfections should be much less than the parent ones, thus Kahr concludes that the crystals "were not faithful enough to store and transfer information from one generation to the next".[93][94]
Gold's "Deep-hot biosphere" model
In the 1970s, Thomas Gold proposed the theory that life first developed not on the surface of the Earth, but several kilometers below the surface. The discovery in the late 1990s of nanobes (filamental structures that are smaller than bacteria, but that may contain DNA) in deep rocks [95] might be seen as lending support to Gold's theory.
It is now reasonably well established that microbial life is plentiful at shallow depths in the Earth, up to 5 kilometres (3.1 mi) below the surface,[95] in the form of extremophile archaea, rather than the better-known eubacteria (which live in more accessible conditions). It is claimed that discovery of microbial life below the surface of another body in our solar system would lend significant credence to this theory. Thomas Gold also asserted that a trickle of food from a deep, unreachable, source is needed for survival because life arising in a puddle of organic material is likely to consume all of its food and become extinct. Gold's theory is that flow of food is due to out-gassing of primordial methane from the Earth's mantle; more conventional explanations of the food supply of deep microbes (away from sedimentary carbon compounds) is that the organisms subsist on hydrogen released by an interaction between water and (reduced) iron compounds in rocks.
"Primitive" extraterrestrial life
An alternative to Earthly abiogenesis is the hypothesis that primitive life may have originally formed extraterrestrially, either in space or on a nearby planet (Mars). (Note that exogenesis is related to, but not the same as, the notion of panspermia). A supporter of this theory was Francis Crick.
Organic compounds are relatively common in space, especially in the outer solar system where volatiles are not evaporated by solar heating.[96] Comets are encrusted by outer layers of dark material, thought to be a tar-like substance composed of complex organic material formed from simple carbon compounds after reactions initiated mostly by irradiation by ultraviolet light. It is supposed that a rain of material from comets could have brought significant quantities of such complex organic molecules to Earth.
An alternative but related hypothesis, proposed to explain the presence of life on Earth so soon after the planet had cooled down, with apparently very little time for prebiotic evolution, is that life formed first on early Mars. Due to its smaller size Mars cooled before Earth (a difference of hundreds of millions of years), allowing prebiotic processes there while Earth was still too hot. Life was then transported to the cooled Earth when crustal material was blasted off Mars by asteroid and comet impacts. Mars continued to cool faster and eventually became hostile to the continued evolution or even existence of life (it lost its atmosphere due to low volcanism); Earth is following the same fate as Mars, but at a slower rate.
Neither hypothesis actually answers the question of how life first originated, but merely shifts it to another planet or a comet. However, the advantage of an extraterrestrial origin of primitive life is that life is not required to have evolved on each planet it occurs on, but rather in a single location, and then spread about the galaxy to other star systems via cometary and/or meteorite impact. Evidence to support the hypothesis is scant, but it finds support in recent study of Martian meteorites found in Antarctica and in studies of extremophile microbes.[97] Additional support comes from a recent discovery of a bacterial ecosystem whose energy source is radioactivity.[98]
A 2001 experiment led by Jason Dworkin[99] subjected a frozen mixture of water, methanol, ammonia and carbon monoxide to UV radiation, mimicking conditions found in an extraterrestrial environment. This combination yielded large amounts of organic material that self-organised to form bubbles or micelles when immersed in water. Dworkin considered these bubbles to resemble cell membranes that enclose and concentrate the chemistry of life, separating their interior from the outside world.
The bubbles produced in these experiments were between 10 to 40 micrometres (0.00039 to 0.00157 in), or about the size of red blood cells. Remarkably, the bubbles fluoresced, or glowed, when exposed to UV light. Absorbing UV and converting it into visible light in this way was considered one possible way of providing energy to a primitive cell. If such bubbles played a role in the origin of life, the fluorescence could have been a precursor to primitive photosynthesis. Such fluorescence also provides the benefit of acting as a sunscreen, diffusing any damage that otherwise would be inflicted by UV radiation. Such a protective function would have been vital for life on the early Earth, since the ozone layer, which blocks out the sun's most destructive UV rays, did not form until after photosynthetic life began to produce oxygen.[62]
Extraterrestrial amino acids
Another idea is that amino acids which were formed extra-terrestrially arrived on Earth via comets. In 2009 it was announced by NASA that scientists have identified one of the fundamental chemical buildings blocks of life in a comet for the first time: glycine, an amino acid, was detected in the material ejected from Comet Wild-2 in 2004 and grabbed by NASA's Stardust probe. Tiny grains, just a few thousandths of a millimetre in size, were collected from the comet and returned to Earth in 2006 in a sealed capsule, and distributed among the world's leading astro-biology labs. NASA said in a statement that it took some time for the investigating team, led by Dr Jamie Elsila, to convince itself that the glycine signature found in Stardust's sample bay was genuine and not just Earthly contamination. Glycine has been detected in meteorites before and there are also observations in interstellar gas clouds claimed for telescopes, but the Stardust find is described as a first in cometary material. It is known that prior to the emergence of life on Earth, the early solar system's planets were regularly bombarded by comets. Dr. Carl Pilcher, who leads NASA's Astrobiology Institute commented that "The discovery of glycine in a comet supports the idea that the fundamental building blocks of life are prevalent in space, and strengthens the argument that life in the Universe may be common rather than rare."[100]
Lipid World
This theory postulates that the first self-replicating object was lipid-like.[101] It is known that phospholipids form bilayers in water while under agitation– the same structure as in cell membranes. These molecules were not present on early Earth, however other amphiphilic long chain molecules also form membranes. Furthermore, these bodies may expand (by insertion of additional lipids), and under excessive expansion may undergo spontaneous splitting which preserves the same size and composition of lipids in the two progenies. The main idea in this theory is that the molecular composition of the lipid bodies is the preliminary way for information storage, and evolution led to the appearance of polymer entities such as RNA or DNA that may store information favorably. Still, no biochemical mechanism has been offered to support the Lipid World theory.
Polyphosphates
The problem with most scenarios of abiogenesis is that the thermodynamic equilibrium of amino acid versus peptides is in the direction of separate amino acids. What has been missing is some force that drives polymerization. The resolution of this problem may well be in the properties of polyphosphates.[102][103] Polyphosphates are formed by polymerization of ordinary monophosphate ions PO4−3. Several mechanisms for such polymerization have been suggested. Polyphosphates cause polymerization of amino acids into peptides [citation needed]. They are also logical precursors in the synthesis of such key biochemical compounds as ATP. A key issue seems to be that calcium reacts with soluble phosphate to form insoluble calcium phosphate (apatite), so some plausible mechanism must be found to keep calcium ions from causing precipitation of phosphate. There has been much work on this topic over the years, but an interesting new idea is that meteorites may have introduced reactive phosphorus species on the early Earth.[104]
PAH world hypothesis
Other sources of complex molecules have been postulated, including extraterrestrial stellar or interstellar origin. For example, from spectral analyses, organic molecules are known to be present in comets and meteorites. In 2004, a team detected traces of polycyclic aromatic hydrocarbons (PAH's) in a nebula.[105] Those are the most complex molecules so far found in space. The use of PAH's has also been proposed as a precursor to the RNA world in the PAH world hypothesis.[106] The Spitzer Space Telescope has recently detected a star, HH 46-IR, which is forming by a process similar to that by which the sun formed. In the disk of material surrounding the star, there is a very large range of molecules, including cyanide compounds, hydrocarbons, and carbon monoxide. PAHs have also been found all over the surface of galaxy M81, which is 12 million light years away from the Earth, confirming their widespread distribution in space.[107]
Multiple genesis
Different forms of life may have appeared quasi-simultaneously in the early history of Earth.[108] The other forms may be extinct, leaving distinctive fossils through their different biochemistry (e.g., using arsenic instead of phosphorus), survive as extremophiles, or simply be unnoticed through their being analogous to organisms of the current life tree. Hartman[109] for example combines a number of theories together, by proposing that:
The first organisms were self-replicating iron-rich clays which fixed carbon dioxide into oxalic and other dicarboxylic acids. This system of replicating clays and their metabolic phenotype then evolved into the sulfide rich region of the hotspring acquiring the ability to fix nitrogen. Finally phosphate was incorporated into the evolving system which allowed the synthesis of nucleotides and phospholipids. If biosynthesis recapitulates biopoesis, then the synthesis of amino acids preceded the synthesis of the purine and pyrimidine bases. Furthermore the polymerization of the amino acid thioesters into polypeptides preceded the directed polymerization of amino acid esters by polynucleotides.
Lynn Margulis's endosymbiotic theory suggests that multiple forms of bacteria entered into symbiotic relationship to form the eukaryotic cell. The horizontal transfer of genetic material between bacteria promotes such symbiotic relationships, and thus many separate organisms may have contributed to building what has been recognised as the Last Universal Common Ancestor (LUCA) of modern organisms. James Lovelock's Gaia theory, proposes that such bacterial symbiosis establishes the environment as a system produced by and supportive of life. His arguments strongly weaken the case for life having evolved elsewhere in the solar system.
See also
- Astrochemistry
- Autocatalytic reactions and order creation
- Biogenesis
- Common descent
- Drake equation
- Entropy and life
- History of Earth
- List of independent discoveries
- List of publications in biology
- Mediocrity principle
- Origin of the world's oceans
- Mimivirus
- Planetary habitability
- Rare Earth hypothesis
- Shadow biosphere
- Thermosynthesis
- Zeolite
- John Needham
References
- ^ Miller-Urey Experiment: Amino Acids & The Origins of Life on Earth
- ^ Zimmer C (2009). "Origins. On the origin of eukaryotes". Science. 325 (5941): 666–8. doi:10.1126/science.325_666. PMID 19661396.
{{cite journal}}
: Unknown parameter|month=
ignored (help) - ^ a b Wilde SA, Valley JW, Peck WH, Graham CM (2001). "Evidence from detrital zircons for the existence of continental crust and oceans on the Earth 4.4 Gyr ago". Nature. 409 (6817): 175–8. doi:10.1038/35051550. PMID 11196637.
{{cite journal}}
: Unknown parameter|month=
ignored (help)CS1 maint: multiple names: authors list (link) - ^ Schopf JW, Kudryavtsev AB, Agresti DG, Wdowiak TJ, Czaja AD (2002). "Laser--Raman imagery of Earth's earliest fossils". Nature. 416 (6876): 73–6. doi:10.1038/416073a. PMID 11882894.
{{cite journal}}
: Unknown parameter|month=
ignored (help)CS1 maint: multiple names: authors list (link) - ^ Hayes, John M. (2006). "The carbon cycle and associated redox processes through time". Phil. Trans. R. Soc. B. 361 (1470): 931–950. doi:10.1098/rstb.2006.1840. PMC 1578725. PMID 16754608.
{{cite journal}}
: Unknown parameter|coauthors=
ignored (|author=
suggested) (help) - ^ Archer, Corey (2006). "Coupled Fe and S isotope evidence for Archean microbial Fe(III) and sulfate reduction". Geology. 34 (3): 153–156. doi:10.1130/G22067.1.
{{cite journal}}
: Unknown parameter|coauthors=
ignored (|author=
suggested) (help) - ^ Cavalier-Smith, Thomas (2006). "Introduction: how and when did microbes change the world?". Phil. Trans. R. Soc. B. 361 (1470): 845–50. doi:10.1098/rstb.2006.1847. PMC 1626534. PMID 16754602.
{{cite journal}}
: Unknown parameter|coauthors=
ignored (|author=
suggested) (help) - ^ Summons, Roger E. (2006). "Steroids, triterpenoids and molecular oxygen". Phil. Trans. R. Soc. B. 361 (1470): 951–68. doi:10.1098/rstb.2006.1837. PMC 1578733. PMID 16754609.
{{cite journal}}
: Unknown parameter|coauthors=
ignored (|author=
suggested) (help) - ^ Philip P. Wiener, ed. (1973). "Spontaneous Generation". Dictionary of the History of Ideas. New York: Charles Scribner's Sons.
{{cite book}}
:|access-date=
requires|url=
(help); External link in
(help); Unknown parameter|chapterurl=
|chapterurl=
ignored (|chapter-url=
suggested) (help) - ^ Lennox, James (2001). Aristotle's Philosophy of Biology: Studies in the Origins of Life Science. New York, NY: Cambridge Press. pp. 229–258. ISBN 978-0521659765.
- ^ Balme, D. M. (1962). "Development of Biology in Aristotle and Theophrastus: Theory of Spontaneous Generation". Phronesis: a journal for Ancient Philosophy. 7 (1–2): 91–104. doi:10.1163/156852862X00052.
{{cite journal}}
: Cite has empty unknown parameter:|coauthors=
(help) - ^ Dobell, C. (1960). Antony Van Leeuwenhoek and his little animals. New York: Dover Publications. ISBN 0486605949.
- ^ Template:Http://mathforum.org/dr.math/faq/faq.prob.intro.html
- ^ Template:Http://wiki.riteme.site/wiki/Rare Earth hypothesis
- ^ Template:Http://wiki.riteme.site/wiki/Planetary habitability
- ^ Oparin, Aleksandr I. (1953). Origin of Life. Dover Publications, New York. p. 196. ISBN 0486602133.
- ^ First life on Earth windmillministries.org, Retrieved on 2008-01-18
- ^ "It is often said that all the conditions for the first production of a living organism are now present, which could ever have been present. But if (and oh! what a big if!) we could conceive in some warm little pond, with all sorts of ammonia and phosphoric salts, light, heat, electricity, &c., present, that a proteine compound was chemically formed ready to undergo still more complex changes, at the present day such matter would be instantly devoured or absorbed, which would not have been the case before living creatures were formed." written in 1871, published in Darwin, Francis, ed. 1887. The life and letters of Charles Darwin, including an autobiographical chapter. London: John Murray. Volume 3. p. 18
- ^ Oparin, A. I. (1924) Proiskhozhozhdenie zhizny, Moscow (Translated by Ann Synge in Bernal (1967)), The Origin of Life, Weidenfeld and Nicolson, London, pages 199–234.
- ^ Oparin, A. I. (1952). The Origin of Life. New York: Dover. ISBN 0486495221.
- ^ Bernal, J.D. (1969). Origins of Life. London: Wiedenfeld and Nicholson.
- ^ Bryson, Bill (2004). A short history of nearly everything. London: Black Swan. pp. 300–2. ISBN 0-552-99704-8.
- ^ Morse, J. W. (1998). "Hadean Ocean Carbonate chemistry". Aquatic Geochemistry. 4: 301–19. doi:10.1023/A:1009632230875.
{{cite journal}}
: Unknown parameter|coauthors=
ignored (|author=
suggested) (help) - ^ Sleep, Norman H. (1989). "Annihilation of ecosystems by large asteroid impacts on early Earth". Nature. 342 (6246): 139–142. doi:10.1038/342139a0. PMID 11536616.
{{cite journal}}
: Unknown parameter|coauthors=
ignored (|author=
suggested) (help) - ^ Maher, Kevin A. (1988). "Impact frustration of the origin of life". Nature. 331 (6157): 612–4. doi:10.1038/331612a0. PMID 11536595.
{{cite journal}}
: Unknown parameter|coauthors=
ignored (|author=
suggested) (help) - ^ Orgel, Leslie E. (2004). "Prebiotic adenine revisited: Eutectics and photochemistry". Origins of Life and Evolution of Biospheres. 34: 361–9. doi:10.1023/B:ORIG.0000029882.52156.c2.
- ^ Robertson, Michael P. (1995). "An efficient prebiotic synthesis of cytosine and uracil". Nature. 375 (6534): 772–774. doi:10.1038/375772a0. PMID 7596408.
{{cite journal}}
: Unknown parameter|coauthors=
ignored (|author=
suggested) (help) - ^ Bada, J. L. (1994). "Impact Melting of Frozen Oceans on the Early Earth: Implications for the Origin of Life" (abstract). Proc. Natl. Acad. Sci. U.S.A. 91 (4): 1248–50. doi:10.1073/pnas.91.4.1248. PMC 43134. PMID 11539550.
{{cite journal}}
: Unknown parameter|coauthors=
ignored (|author=
suggested) (help) - ^ "Did Life Evolve in Ice? - Arctic & Antarctic". DISCOVER Magazine. Retrieved 2008-07-03.
- ^ Levy, M. (2000). "Prebiotic synthesis of adenine and amino acids under Europa-like conditions". Icarus. 145 (2): 609–13. doi:10.1006/icar.2000.6365. PMID 11543508.
{{cite journal}}
:|access-date=
requires|url=
(help); Unknown parameter|coauthors=
ignored (|author=
suggested) (help); Unknown parameter|month=
ignored (help) - ^ Trinks, Hauke (2005). "Ice And The Origin Of Life". Origins of Life and Evolution of the Biosphere. 35 (5): 429–45. doi:10.1007/s11084-005-5009-1. PMID 16231207. Retrieved 2008-02-11.
{{cite journal}}
: Unknown parameter|coauthors=
ignored (|author=
suggested) (help); Unknown parameter|month=
ignored (help) - ^ Mojzis, S. J. (1996). "Evidence for life on earth before 3,800 million years ago". Nature. 384 (6604): 55–9. doi:10.1038/384055a0. PMID 8900275.
{{cite journal}}
: Unknown parameter|coauthors=
ignored (|author=
suggested) (help) - ^ Lazcano, A. (1994). "How long did it take for life to begin and evolve to cyanobacteria?". Journal of Molecular Evolution. 39 (6): 546–54. doi:10.1007/BF00160399. PMID 11536653.
{{cite journal}}
: Unknown parameter|coauthors=
ignored (|author=
suggested) (help) - ^ Gibson, DG; Glass, JI; Lartigue, C; Noskov, VN; Chuang, RY; Algire, MA; Benders, GA; Montague, MG; Ma, L (2010). "Creation of a Bacterial Cell Controlled by a Chemically Synthesized Genome". Science (New York, N.Y.). 329 (5987). Science (journal): 52–6. doi:10.1126/science.1190719. PMID 20488990.
- ^ "Scientists Create First Self-Replicating Synthetic Life".
- ^ Bernal, John Desmond (1949). "The Physical Basis of Life". Proceedings of the Physical Society. Section A, 1949. 62: 537–538. doi:10.1088/0370-1298/62/9/301.
{{cite journal}}
: Cite has empty unknown parameter:|coauthors=
(help) - ^ Chyba, Christopher (1992). "Endogenous production, exogenous delivery and impact-shock synthesis of organic molecules: an inventory for the origins of life". Nature. 355 (6356): 125–32. doi:10.1038/355125a0. PMID 11538392.
{{cite journal}}
: Unknown parameter|coauthors=
ignored (|author=
suggested) (help) - ^ Shapiro, Robert (1987). Origins: A Skeptic's Guide to the Creation of Life on Earth. Bantam Books. p. 110. ISBN 0671459392.
- ^ Miller, Stanley L. (1953). "A Production of Amino Acids Under Possible Primitive Earth Conditions". Science. 117 (3046): 528–9. doi:10.1126/science.117.3046.528. PMID 13056598.
{{cite journal}}
: Cite has empty unknown parameter:|coauthors=
(help) - ^ Oró, J. (1961). "Mechanism of synthesis of adenine from hydrogen cyanide under possible primitive Earth conditions". Nature. 191: 1193–4. doi:10.1038/1911193a0. PMID 13731264.
- ^ Menor-Salván C, Ruiz-Bermejo DM, Guzmán MI, Osuna-Esteban S, Veintemillas-Verdaguer S (2007). "Synthesis of pyrimidines and triazines in ice: implications for the prebiotic chemistry of nucleobases". Chemistry. 15 (17) (17): 4411–8. doi:10.1002/chem.200802656. PMC 19288488. PMID 19288488.
{{cite journal}}
: Check|pmc=
value (help)CS1 maint: multiple names: authors list (link) - ^ Experiments on origin of organic molecules Nitro.biosci.arizona.edu, Retrieved on 2008-01-13
- ^ Schuster, P.; Eigen, M. (1979). The hypercycle, a principle of natural self-organization. Berlin: Springer-Verlag. ISBN 0-387-09293-5.
{{cite book}}
: CS1 maint: multiple names: authors list (link) - ^ origin of life thebioreview.com Retrieved on 2008-01-14
- ^ Hoffmann, G. W. (1974). "On the Origin of the Genetic Code and the Stability of the Translation Apparatus". J. Mol. Biol. Vol. 86. pp. 349–362.
- ^ Orgel, L. (1963). "The Maintenance of the Accuracy of Protein Synthesis and its Relevance to Ageing". Proc. Nat. Acad. Sci. USA. Vol. 49. pp. 517–521.
- ^ Hoffmann, G. W. (1975). H. Eyring (ed.). "The Stochastic Theory of the Origin of Life". Annual Review of Physical Chemistry. Vol. 26. pp. 123–144.
- ^ Huber, C. (1998). "Peptides by activation of amino acids with CO on (Ni,Fe)S surfaces: implications for the origin of life". Science. 281 (5377): 670–2. doi:10.1126/science.281.5377.670. PMID 9685253.
{{cite journal}}
: Unknown parameter|coauthors=
ignored (|author=
suggested) (help) - ^ Dartnell, Lewis (2008-01-12). "Life's a beach on planet Earth". New Scientist.
{{cite news}}
: Cite has empty unknown parameter:|coauthors=
(help); Unknown parameter|curly=
ignored (help) - ^ Adam, Zachary (2007). "Actinides and Life's Origins". Astrobiology. 7 (6): 852–72. doi:10.1089/ast.2006.0066. PMID 18163867.
{{cite journal}}
: Cite has empty unknown parameter:|coauthors=
(help) - ^ Parnell, John (2004). "Mineral Radioactivity in Sands as a Mechanism for Fixation of Organic Carbon on the Early Earth" (PDF). Origins of Life and Evolution of Biospheres. 34 (6): 533–547. doi:10.1023/B:ORIG.0000043132.23966.a1.
- ^ Michaelian, Karo (2009). "Thermodynamic Function of Lfe" (PDF). ArXiv.
- ^ Cnossen, I.; Sanz-Forcada, Jorge; Favata, Fabio; Witasse, Olivier; Zegers, Tanja; Arnold, Neil F.; et al. (2007). "The habitat of early life: Solar X-ray and UV radiation at Earth's surface 4-3.5 billion years ago". J. Geophys. Research. 112: E02008. doi:10.1029/2006JE002784.
{{cite journal}}
:|format=
requires|url=
(help); Explicit use of et al. in:|last=
(help) - ^ a b Powner MW, Gerland B, Sutherland JD (2009). "Synthesis of activated pyrimidine ribonucleotides in prebiotically plausible conditions". Nature. 459 (7244): 239–42. doi:10.1038/nature08013. PMID 19444213.
{{cite journal}}
: Unknown parameter|month=
ignored (help)CS1 maint: multiple names: authors list (link) - ^ Lowe, D. R. and Tice, M. M, Donald R.; Tice, Michael M. (2004). "Geologic evidence for Archean atmospheric and climatic evolution: Fluctuating levels of CO2, CH4, and O2 with an overriding tectonic control". Geology. 32: 493–496. doi:10.1130/G20342.1.
{{cite journal}}
:|format=
requires|url=
(help)CS1 maint: multiple names: authors list (link) - ^ Michaelian, Karo (2010). "Thermodynamic Origin of Lfe" (PDF). Earth Syst. Dynam. Discuss. 1: 1–39. doi:10.5194/esdd-1-1-2010.
{{cite journal}}
: CS1 maint: unflagged free DOI (link) - ^ Michaelian, Karo (2009). "Thermodynamic Origin of Lfe" (PDF). ArXiv.
- ^ Michaelian, Karo (2010). "Homochirality Through Photon-Induced Melting Of RNA/DNA: Thermodynamic Dissipation Theory Of The Origin Of Life" (PDF). WebmedCentral Biochemistry. 1: WMC00924.
{{cite journal}}
: line feed character in|title=
at position 48 (help) - ^ Noyes HP, Bonner WA, Tomlin JA (1977). "On the origin of biological chirality via natural beta-decay". Orig. Life. 8 (1): 21–3. doi:10.1007/BF00930935. PMID 896189.
{{cite journal}}
: Unknown parameter|month=
ignored (help)CS1 maint: multiple names: authors list (link) - ^ Hazen, Robert M. (2005). Genesis: the scientific quest for life's origin. Washington, D.C: Joseph Henry Press. ISBN 0-309-09432-1.
- ^ Clark, S. (1999). "Polarised starlight and the handedness of Life". American Scientist. 97: 336–43. doi:10.1511/1999.4.336.
- ^ a b Mullen L (September 5, 2005). "Building Life from Star-Stuff". Astrobiology Magazine.
- ^ Martin, William (2003). "On the origins of cells: a hypothesis for the evolutionary transitions from abiotic geochemistry to chemoautotrophic prokaryotes, and from prokaryotes to nucleated cells". Phil. Trans. R. Soc. B. 358 (1429): 59–85. doi:10.1098/rstb.2002.1183. PMC 1693102. PMID 12594918.
{{cite journal}}
: Unknown parameter|coauthors=
ignored (|author=
suggested) (help) - ^ Koonin EV, Senkevich TG, Dolja VV (2006). "The ancient Virus World and evolution of cells". Biol. Direct. 1: 29. doi:10.1186/1745-6150-1-29. PMC 1594570. PMID 16984643. Retrieved 2008-10-20.
{{cite journal}}
: More than one of|pages=
and|page=
specified (help)CS1 maint: multiple names: authors list (link) CS1 maint: unflagged free DOI (link) - ^ Vlassov AV, Kazakov SA, Johnston BH, Landweber LF (2005). "The RNA world on ice: a new scenario for the emergence of RNA information". J. Mol. Evol. 61 (2): 264–73. doi:10.1007/s00239-004-0362-7. PMID 16044244.
{{cite journal}}
:|access-date=
requires|url=
(help); Unknown parameter|month=
ignored (help)CS1 maint: multiple names: authors list (link) - ^ Nussinov, Mark D. (1997). "Emerging Concepts of Self-organization and the Living State". Biosystems. 42 (2–3): 111–118. doi:10.1016/S0303-2647(96)01699-1. PMID 9184757. Retrieved 2009-08-02.
{{cite journal}}
: Unknown parameter|coauthors=
ignored (|author=
suggested) (help) - ^ Ma W, Yu C, Zhang W, Hu J (2007). "Nucleotide synthetase ribozymes may have emerged first in the RNA world". RNA (New York, N.Y.). 13 (11): 2012–9. doi:10.1261/rna.658507. PMC 2040096. PMID 17878321.
{{cite journal}}
: Unknown parameter|month=
ignored (help)CS1 maint: multiple names: authors list (link) - ^ Johnston, W. K. (2001). "RNA-Catalyzed RNA Polymerization: Accurate and General RNA-Templated Primer Extension". Science. 292 (5520): 1319–1325. doi:10.1126/science.1060786. PMID 11358999.
{{cite journal}}
: Unknown parameter|coauthors=
ignored (|author=
suggested) (help) - ^ Szostak, Jack W. (June 4, 2008). "The Origins of Function in Biological Nucleic Acids, Proteins, and Membranes". HHMI. Retrieved 2008-11-29.
- ^ Lincoln, Tracey A. (January 8, 2009). "Self-Sustained Replication of an RNA Enzyme". Science. 323 (5918). New York: American Association for the Advancement of Science: 1229–32. doi:10.1126/science.1167856. ISSN 1095-9203. PMC 2652413. PMID 19131595. Retrieved 2009-01-13.
{{cite journal}}
: Unknown parameter|coauthors=
ignored (|author=
suggested) (help) - ^ Orgel, L. (1994). "The origin of life on earth". Scientific American. 271 (4): 81.
{{cite journal}}
: Cite has empty unknown parameter:|coauthors=
(help) - ^ Levy, Matthew (1998). "The stability of the RNA bases: Implications for the origin of life". PNAS. 95 (14): 7933–7938. doi:10.1073/pnas.95.14.7933. PMC 20907. PMID 9653118.
{{cite journal}}
: Unknown parameter|coauthors=
ignored (|author=
suggested) (help) - ^ Larralde, R. (1995). "Rates of Decomposition of Ribose and Other Sugars: Implications for Chemical Evolution". PNAS. 92 (18): 8158–8160. doi:10.1073/pnas.92.18.8158. PMC 41115. PMID 7667262.
{{cite journal}}
: Unknown parameter|coauthors=
ignored (|author=
suggested) (help) - ^ Lindahl, Tomas (1993). "Instability and decay of the primary structure of DNA". Nature. 362 (6422): 709–715. doi:10.1038/362709a0. PMID 8469282.
{{cite journal}}
: Cite has empty unknown parameter:|coauthors=
(help) - ^ Orgel, Leslie (2000). "A Simpler Nucleic Acid". Science. 290 (5495): 1306–1307. doi:10.1126/science.290.5495.1306. PMID 11185405.
{{cite journal}}
: Cite has empty unknown parameter:|coauthors=
(help) - ^ Nelson, K. E. (2000). "Peptide nucleic acids rather than RNA may have been the first genetic molecule" (abstract). PNAS. 97 (8): 3868–3871. doi:10.1073/pnas.97.8.3868. PMC 18108. PMID 10760258.
{{cite journal}}
: Unknown parameter|coauthors=
ignored (|author=
suggested) (help) - ^ Anastasi C, Crowe MA, Powner MW, Sutherland JD (2006). "Direct Assembly of Nucleoside Precursors from Two- and Three-Carbon Units". Angewandte Chemie International Edition. 45 (37): 6176–9. doi:10.1002/anie.200601267. PMID 16917794.
{{cite journal}}
: CS1 maint: multiple names: authors list (link) - ^ Powner MW, Sutherland JD (2008). "Potentially Prebiotic Synthesis of Pyrimidine β-D-Ribonucleotides by Photoanomerization/Hydrolysis of α-D-Cytidine-2-Phosphate". ChemBioChem. 9 (15): 2386–7. doi:10.1002/cbic.200800391. PMID 18798212.
- ^ Huang W, Ferris JP (2006). "One-step, regioselective synthesis of up to 50-mers of RNA oligomers by montmorillonite catalysis". J. Am. Chem. Soc. 128 (27): 8914–9. doi:10.1021/ja061782k. PMID 16819887.
{{cite journal}}
: Unknown parameter|month=
ignored (help) - ^ Orgel LE (2000). "Self-organizing biochemical cycles". Proc. Natl. Acad. Sci. U.S.A. 97 (23): 12503–7. doi:10.1073/pnas.220406697. PMC 18793. PMID 11058157.
{{cite journal}}
: Unknown parameter|month=
ignored (help) - ^ Muller, A.W.J. (1985). "Thermosynthesis by biomembranes: energy gain from cyclic temperature changes". Journal of Theoretical Biology. 115: 319–321.
- ^ Muller, A.W.J. (1995). "Were the first organisms heat engines? A new model for biogenesis and the early evolution of biological energy conversion". Progress in Biophysics and Molecular Biology. 63 (2): 193–231. doi:10.1016/0079-6107(95)00004-7. PMID 7542789.
- ^ Freeman Dyson (1985). Origins of Life. Cambridge: Cambridge University Press. ISBN 0521626684.
- ^ Muller, A.W.J. (2005). "Thermosynthesis as energy source for the RNA World: a model for the bioenergetics of the origin of life". Biosystems. 82 (1): 93–102. doi:10.1016/j.biosystems.2005.06.003. PMID 16024164.
- ^
Sun, F.J. and Caetano-Anolles, G. (2008). "The origin and evolution of tRNA inferred from phylogenetic analysis of structure". Journal of Molecular Evolution. 66 (1): 21–35. doi:10.1007/s00239-007-9050-8. PMID 18058157.
{{cite journal}}
: CS1 maint: multiple names: authors list (link) - ^
Muller, A.W.J. ; Schulze-Makuch, D. (2006). "Sorption heat engines: simple inanimate negative entropy generators". Physica A. 362: 369–381. doi:10.1016/j.physa.2005.12.003.
{{cite journal}}
: CS1 maint: multiple names: authors list (link) - ^
Orgel, L. (1987). "Evolution of the genetic apparatus: a review". Cold Spring Harbor Symposia on Quantitative Biology. 52: 9–16. doi:10.1101/sqb.1987.052.01.004. PMID 2456886.
{{cite journal}}
: Unknown parameter|doi_brokendate=
ignored (|doi-broken-date=
suggested) (help) - ^ Panno, Joseph (2005). The cell: evolution of the first organism. New York: Facts on File. ISBN 0-8160-4946-7.
- ^ publications
- ^ Stuart Kauffman (1993). The Origins of Order: Self-Organization and Selection in Evolution (Chapter 7). Oxford University Press. ISBN 978-0-19-507951-7
- ^ Dawkins, Richard (1996) [1986]. The Blind Watchmaker. New York: W. W. Norton & Company, Inc. pp. 148–161. ISBN 0-393-31570-3.
- ^ Cairns-Smith, A. G. (1982). Genetic takeover and the mineral origins of life. Cambridge, UK: Cambridge University Press. ISBN 0-521-23312-7.
- ^ Bullard T, Freudenthal J, Avagyan S, Kahr B (2007). "Test of Cairns-Smith's crystals-as-genes hypothesis". Faraday Discuss. 136: 231–45. doi:10.1039/b616612c.
{{cite journal}}
: CS1 maint: multiple names: authors list (link) - ^ Caroline Moore (16 July 2007). "Crystals as genes?". Chemical Science.
- ^ a b Nanobes–Intro microscopy-uk.org, Retrieved on 2008-01-14
- ^ Chang, Kenneth (2009-08-18). "From a Distant Comet, a Clue to Life". Space & Cosmos. New York Times. p. A18. Retrieved 2009-08-19.
- ^ Tough Earth bug may be from Mars - 25 September 2002 - New Scientist
- ^ Lin, Li-Hung (2006). "Long-Term Sustainability of a High-Energy, Low-Diversity Crustal Biome". Science. 314 (5798): 479–482. doi:10.1126/science.1127376. PMID 17053150. 5798.
{{cite journal}}
: Unknown parameter|coauthors=
ignored (|author=
suggested) (help); Unknown parameter|month=
ignored (help) - ^ Jason P. Dworkin (January 30, 2001). "Self-assembling amphiphilic molecules: Synthesis in simulated interstellar/precometary ices". Proc. Nat. Acad. Sciences. 98 (3): 815–819. doi:10.1073/pnas.98.3.815. PMC 14665. PMID 11158552.
{{cite journal}}
: Unknown parameter|coauthors=
ignored (|author=
suggested) (help) - ^ "'Life chemical' detected in comet". BBC News. August 18, 2009.
- ^ Origin of Life at the Weizmann Institute
- ^ Brown MR, Kornberg A (2004). "Inorganic polyphosphate in the origin and survival of species". Proc. Natl. Acad. Sci. U.S.A. 101 (46): 16085–7. doi:10.1073/pnas.0406909101. PMC 528972. PMID 15520374.
{{cite journal}}
: Unknown parameter|month=
ignored (help) - ^ The Origin Of Life
- ^ Pasek MA (2008). "Rethinking early Earth phosphorus geochemistry". Proc. Natl. Acad. Sci. U.S.A. 105 (3): 853–8. doi:10.1073/pnas.0708205105. PMC 2242691. PMID 18195373.
{{cite journal}}
: Unknown parameter|month=
ignored (help) - ^ Witt AN, Vijh UP, Gordon KD (2003). "Discovery of Blue Fluorescence by Polycyclic Aromatic Hydrocarbon Molecules in the Red Rectangle". Bulletin of the American Astronomical Society. 35: 1381.
{{cite journal}}
: CS1 maint: multiple names: authors list (link) - ^ Battersby, S. (2004). Space molecules point to organic origins. Retrieved January 11, 2004 from http://www.newscientist.com/article/dn4552-space-molecules-point-to-organic-origins.html
- ^ Astrobiology Magazine [1] Accessed 26 April 2008
- ^ Are Aliens Among Us? In pursuit of evidence that life arose on Earth more than once, scientists are searching for microbes that are radically different from all known organisms Scientific American. 19 November 2007
- ^ Hartman, Hyman (1998) "Photosynthesis and the Origin of Life" (Origins of Life and Evolution of Biospheres, Volume 28, Numbers 4–6 / October, 1998)
Further reading
- Arrhenius, Gustaf (1997). "Entropy and Charge in Molecular Evolution—the Case of Phosphate". Journal of Theoretical Biology. 187 (4): 503–522. doi:10.1006/jtbi.1996.0385. PMID 9299295.
{{cite journal}}
: Unknown parameter|coauthors=
ignored (|author=
suggested) (help) - Buehler, Lukas K. (2000–2005) The physico-chemical basis of life, http://www.whatislife.com/about.html accessed 27 October 2005.
- Davies, Paul (1998). The Fifth Miracle. Penguin Science, London. ISBN 0-140-28226-2.
- De Duve, Christian (1996). Vital Dust: The Origin and Evolution of Life on Earth. Basic Books. ISBN 0-465-09045-1.
{{cite book}}
: Unknown parameter|month=
ignored (help) - Fernando CT, Rowe, J (2007). "Natural selection in chemical evolution". Journal of Theoretical Biology. 247 (1): 152–67. doi:10.1016/j.jtbi.2007.01.028. PMID 17399743.
{{cite journal}}
: CS1 maint: multiple names: authors list (link) - Hartman, Hyman (1998). "Photosynthesis and the Origin of Life". Origins of Life and Evolution of Biospheres. 28 (4–6): 515–521. doi:10.1023/A:1006548904157.
{{cite journal}}
: Cite has empty unknown parameter:|coauthors=
(help) - Harris, Henry (2002). Things come to life. Spontaneous generation revisited. Oxford: Oxford University Press. ISBN 0198515383.
{{cite book}}
: Cite has empty unknown parameter:|coauthors=
(help) - Hazen, Robert M. (2005). Genesis: The Scientific Quest for Life's Origins. Joseph Henry Press. ISBN 0-309-09432-1.
{{cite book}}
: Unknown parameter|month=
ignored (help) - Gribbon, John (1998). The Case of the Missing Neutrino's and other Curious Phenomena of the Universe. Penguin Science, London. ISBN 0-140-28734-5.
- Horgan, J (1991). "In the beginning". Scientific American. 264: 100–109. (Cited on p. 108).
- Huber, C. and Wächterhäuser, G., (1998). "Peptides by activation of amino acids with CO on (Ni,Fe)S surfaces: implications for the origin of life". Science. 281 (5377): 670–672. doi:10.1126/science.281.5377.670. PMID 9685253.
{{cite journal}}
: CS1 maint: extra punctuation (link) CS1 maint: multiple names: authors list (link) (Cited on p. 108). - Knoll, Andrew H. (2003). Life on a Young Planet: The First Three Billion Years of Evolution on Earth. Princeton University Press. ISBN 0691009783.
- Luisi, Pier Luigi (2006). The Emergence of Life: From Chemical Origins to Synthetic Biology. Cambridge University Press. ISBN 0521821177.
- Martin, W. and Russell M.J. (2002). "On the origins of cells: a hypothesis for the evolutionary transitions from abiotic geochemistry to chemoautotrophic prokaryotes, and from prokaryotes to nucleated cells". Philosophical Transactions of the Royal Society: Biological sciences. 358 (1429): 59–85. doi:10.1098/rstb.2002.1183. PMC 1693102. PMID 12594918.
- Maynard Smith, John (2000-03-16). The Origins of Life: From the Birth of Life to the Origin of Language. Oxford Paperbacks. ISBN 0-19-286209-X.
{{cite book}}
: Unknown parameter|coauthors=
ignored (|author=
suggested) (help) - Morowitz, Harold J. (1992) "Beginnings of Cellular Life: Metabolism Recapitulates Biogenesis". Yale University Press. ISBN 0-300-05483-1
- NASA Astrobiology Institute: Earth's Early Environment and Life
- NASA Specialized Center of Research and Training in Exobiology: Gustaf O. Arrhenius
- Pitsch, Stefan (2000). "Concentration of Simple Aldehydes by Sulfite-Containing Double-Layer Hydroxide Minerals: Implications for Biopoesis" (abstract). Helvetica Chimica Acta. 83 (9): 2398 2411. doi:10.1002/1522-2675(20000906)83:9<2398::AID-HLCA2398>3.0.CO;2-5.
{{cite journal}}
: Unknown parameter|coauthors=
ignored (|author=
suggested) (help) - Russell MJ, Hall AJ, Cairns-Smith AG, Braterman PS (1988). "Submarine hot springs and the origin of life". Nature. 336: 117. doi:10.1038/336117a0.
{{cite journal}}
: More than one of|pages=
and|page=
specified (help)CS1 maint: multiple names: authors list (link) - Dedicated issue of Philosophical Transactions B on Major Steps in Cell Evolution freely available.
- Dedicated issue of Philosophical Transactions B on the Emergence of Life on the Early Earth freely available.
External links
- The Deep Hot Biosphere Theory - Thomas Gold, article from PNAS Proc. Natl. Acad. Sci. USA Vol. 89, pp. 6045-6049, July 1992 Microbiology
- The Origin of Life Video by John Maynard Smith
- "Harvard Team Creates the World's 1st Synthesized Cells"
- Template:PDFlink
- Martin A. Nowak and Hisashi Ohtsuki.Prevolutionary dynamics and the origin of evolution. Proceedings of the National Academy of Sciences 2008
- "Exploring Life's Origins: a Virtual Exhibit"
- "SELF-REPLICATION: Even peptides do it" by Stuart A. Kauffman (web archive version as original page no longer accessible)
- Origins of Life website including papers, resources, by Dr. Michael Russell at the U. of Glasgow
- Possible Connections Between Interstellar Chemistry and the Origin of Life on the Earth
- Scientists Find Clues That Life Began in Deep Space—NASA Astrobiology Institute
- Self-organizing biochemical cycles—by Leslie Orgel
- How Life Began: New Research Suggests Simple Approach
- Primordial Soup's On: Scientists Repeat Evolution's Most Famous Experiment–an article in Scientific American. March 28, 2007
- Illustrations from Evolution (textbook)
- An abiogenesis primer for laymen